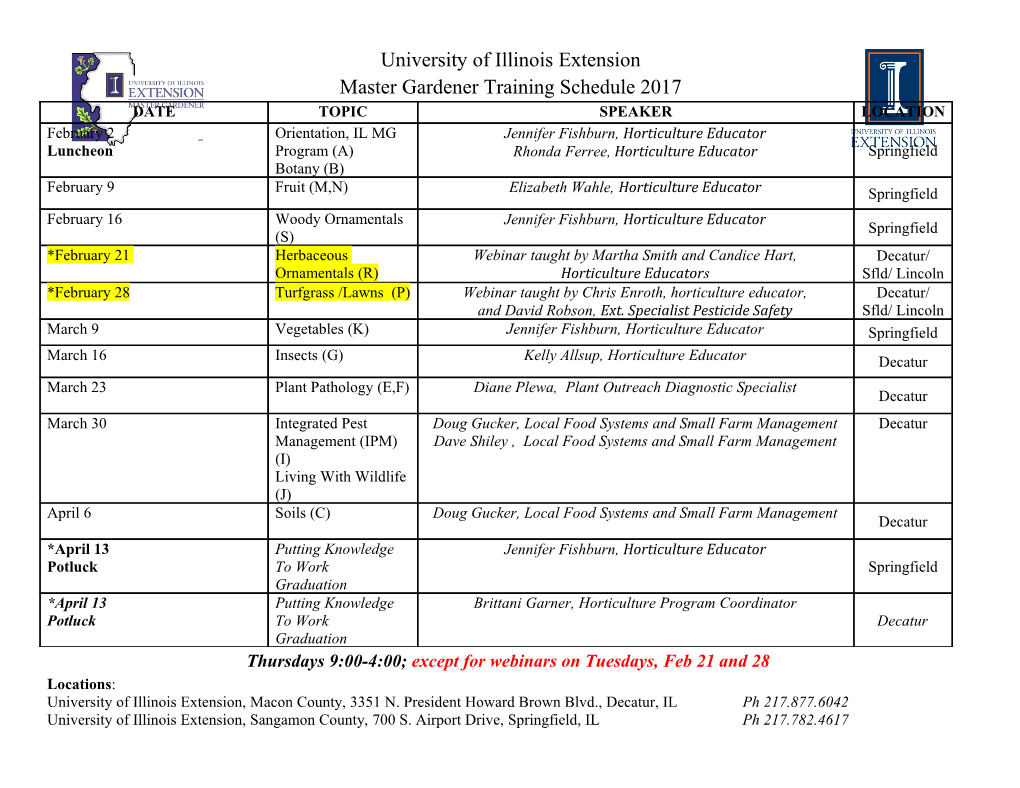
Elucidating the Role of Nanostructured Pigment Granules in the Dynamic Coloration of Cephalopods: A Pathway Towards New Bioinspired Materials by Thomas L. Williams B.A. in Chemistry, Colby College A dissertation submitted to The Faculty of the College of Science of Northeastern University in partial fulfillment of the requirements for the degree of Doctor of Philosophy February 14th, 2019 Dissertation directed by Leila Deravi Professor of Chemistry and Chemical Biology 1 Acknowledgements First, I would like to sincerely extend my gratitude to my advisor, Prof. Leila Deravi, for an incredible amount of direction, advice, and patience. I would also like to thank my dissertation committee members: Profs. Steven Lopez, Eugene Smotkin, and Rudi Seitz. Each of you have contributed significantly to this work and to my growth as a chemist through your comments and guidance. Thanks to my family, who have always been supportive of my academic endeavors. I also need to extend my thanks to all the current and previous members of the Biomaterials Design Group, especially Christopher Dibona, Dr. Sean Dinneen, Dr. Amrita Kumar, and Camille Martin, aka Team Pigment, for their assistance, advice and support. I would also like to thank Conor Gomes, Cassandra Martin, and Dr. Jeff Paten for their insight and friendship. I would also like to thank Northeastern University and the Department of Chemistry and Chemical Biology for the opportunity to work and study here. I would like to specifically thank Prof. Jared Auclair and Dr. John de la Parra for their assistance with mass spectrometry, as well as Prof. Jason Guo, for his assistance with NMR. I also am grateful to Emanuelle Hestermann, Paige Scannell, Rich Pumphrey and Tara Loschiavo. Thanks to the faculty and staff at the Marine Biological Laboratory, especially Dr. Roger Hanlon and Dr. Stephen Senft, for their collaboration and hospitality. Without their work, my own would lack biological context. I would also like to thank my friends, coworkers, and mentors at the University of New Hampshire. Finally, thanks for funding goes to the National Science Foundation (DMR- 1700720), the Barnett Institute of Chemical and Biological Analysis, and the Department of Chemistry and Chemical Biology at Northeastern University. 2 Abstract of Dissertation Cephalopods such as squid, octopus, and cuttlefish can quickly and accurately modulate their coloration in order to camouflage themselves to avoid predators, hunt prey, or communicate within their natural environment. This ability is made possible, in part, by chromatophores: pigmentary organs that can expand or relax to impart a global color change to the animal. While it is known that visible color originates from the nanostructured pigment granules that populate the chromatophore, their compositional and structural contributions to coloration remain largely unknown. The overarching goal of this dissertation is to elucidate the structure and function of the cephalopod chromatophore pigment granules as a means to inspire the design of new advanced optical materials. First, the presence of ommochrome pigments within the chromatophore granules was confirmed, and these pigments were identified as xanthommatin and decarboxylated xanthommatin. In this process, the pigments were extracted from the granules of isolated squid Doryteuthis pealeii chromatophores, resulting in a 70.8% decrease in the diameter of the granules, as well as a loss of absorbance, suggesting that non-pigmentary structural components, likely proteins, contribute to granule structure as well. In the same study, we examined the effect of granule structure on the optical properties of the pigments, finding that the extracted pigment features a blue shift in maximum absorbance as well as an altered fluorescence profile compared to the intact granules, suggesting that the optical properties of chromatophore pigment granules arise from an interaction between the pigmentary and structural components. Next, we performed a proteomic study of chromatophores and pigment granules isolated from D. pealeii dermal tissue to determine what proteins are in the granules that may also 3 contribute to coloration. Lens crystallin proteins, specifically Ω- and S-crystallin isoforms, were found in the pigment granules, suggesting that the interaction between the pigment and these high- refractive index proteins may enhance absorbance through scattering effects. Reflectin, a cephalopod-specific protein responsible for structural coloration, was also identified in chromatophores, but not within the pigment granules. This finding inspired a re-examination of the chromatophore, leading to the conclusion that these organs incorporate elements of protein- based structural coloration, likely through thin-film interference that may amplify the colors displayed by the animal, originating from reflectin in sheath cells in the periphery of the chromatophore. Finally, a new, scalable method for the synthesis of xanthommatin, the primary pigment confined to the chromatophore granules, was developed and characterized. By replacing the traditionally used oxidizing agent with an applied electrochemical potential, the reaction becomes simpler while retaining its kinetics, specificity, and yield. Computational chemistry, informed by previous literature, was used to determine the mechanisms underlying the reaction so that it can more easily be applied to the synthesis of xanthommatin derivatives. Together, these results indicate a possible pathway through which the production of asymmetric phenoxazinones might be scaled up for future materials applications. 4 Table of Contents Acknowledgements 2 Abstract of Dissertation 3 Table of Contents 5 List of Figures 7 List of Tables 8 Chapter 1: Introduction to Cephalopod-Inspired Materials 9 1.1 Bioinspired Design 9 1.2 Cephalopods and Dynamic Coloration 11 1.3 Iridophores 13 1.4 Leucophores 19 1.5 Chromatophores 21 1.6 Ommochromes and Xanthommatin 26 1.7 Pigment Granules in Insects 31 1.8 Reflectin and Crystallin 33 1.9 Cephalopod Inspired Materials 37 1.10 Dissertation Aims 42 Chapter 2: Overview of Methods 44 2.1 Animal Collection 44 2.2 Chromatophore Isolation 44 2.3 Collection of Chromatophore Granules 44 2.4 Pigment Extraction 45 2.5 Pigment Separation 45 2.6 Scanning Electron Microscopy 46 2.7 Ultraviolet-Visible Light Spectroscopy 46 2.8 Fluorescence Spectroscopy 47 2.9 Mass Spectrometry 47 2.10 Isolation of Chromatophores for Whole Cell Analysis 48 2.11 Protein Purification and Analysis Using Mass Spectrometry 48 2.12 Sample Collection and RNA Sequencing of Squid Chromatophore 50 2.13 Transcriptome Assembly 51 2.14 Protein Identification using BLAST 51 2.15 Spectral Count Data Processing 52 2.16 Synthesis of Xanthommatin 53 2.17 Electrosynthesis of Xanthommatin 53 2.18 Characterization of Electrosynthesized Xanthommatin 54 2.19 Confirmation of Electrosynthesized Xanthommatin Structure 54 2.20 Computational Methods 55 5 Chapter 3: Contributions of Phenoxazone-Based Pigments to the Structure 53 and Function of Nanostructured Granules in Squid Chromatophores 3.1 Introduction 53 3.2 Results and Discussion 58 3.3 Conclusions 68 Chapter 4: Dynamic Pigmentary and Structural Coloration Within 70 Cephalopod Chromatophore Organs 4.1 Introduction 70 4.2 Results 4.2.1. Expanded Chromatophores Exhibit Intense Structural Color 72 4.2.2 Composition Analysis of the Squid Chromatophore 74 4.2.3. Crystallin But Not Reflectin Is Found Amidst Pigment Granules 78 4.2.4. Reflectin Molecules Are Distributed Throughout Sheath Cells 80 4.2 Discussion 83 Chapter 5: A scalable method to synthesize the xanthommatin biochrome 89 via an electro catalyzed oxidation of tryptophan metabolites 5.1 Introduction 89 5.2 Results 91 5.3 Conclusions 101 Chapter 6: Conclusions and Recommendations 102 References 105 Appendices 117 A1. Proteomics Data from Chromatophores and Pigment Granules 117 A2. Computational Data 159 A2.1. Coordinates and energies of the closed-shell substitution reaction 159 A2.2. Coordinates and energies of the open-shell substitution reaction 163 A2.3. Coordinates and energies of the closed-shell addition reaction 166 A2.4. Coordinates and energies of the open-shell addition reaction 171 A2.5. Coordinates and energies of the final product, H2Xa 175 A2.6. Coordinates and energies of the leaving groups 178 6 List of Figures Figure 1.1. Photographs of cephalopods exhibiting dynamic coloration 11 Figure 1.2. Male cuttlefish performing the Intense Zebra Display 12 Figure 1.3. Cross-sectional TEM Image of Octopus dofleini dermis 14 Figure 1.4. Multilayer interference diagram and simulated effect 16 Figure 1.5. Illustration of tunable iridophore mechanism 18 Figure 1.6. Reflectance spectra and images of switchable iridophores 20 Figure 1.7. Drawing of cephalopod chromatophore organ 22 Figure 1.8. Examples of ommochromes derived from xanthommatin 26 Figure 1.9. Biochemical synthesis of xanthommatin 29 Figure 1.10. Kynurenine monooxygenase distribution in nature 31 Figure 1.11. Proposed model of ommochromosome formation in spiders 33 Figure 1.12. Repeated peptides in and overall composition of reflectin 36 Figure 1.13. Examples of cephalopod inspired technology 40 Figure 2.1 SDS-PAGE of proteins collected from whole chromatophores 49 Figure 2.2 SDS-PAGE of proteins from isolated granules, pigment, and 50 extracted granules Figure 3.1. Results of pigment extraction from granules 59 Figure 3.2. Separation of pigments by preparative TLC 61 Figure 3.3. 3D Fluorescence spectra of granules and pigments 63
Details
-
File Typepdf
-
Upload Time-
-
Content LanguagesEnglish
-
Upload UserAnonymous/Not logged-in
-
File Pages178 Page
-
File Size-