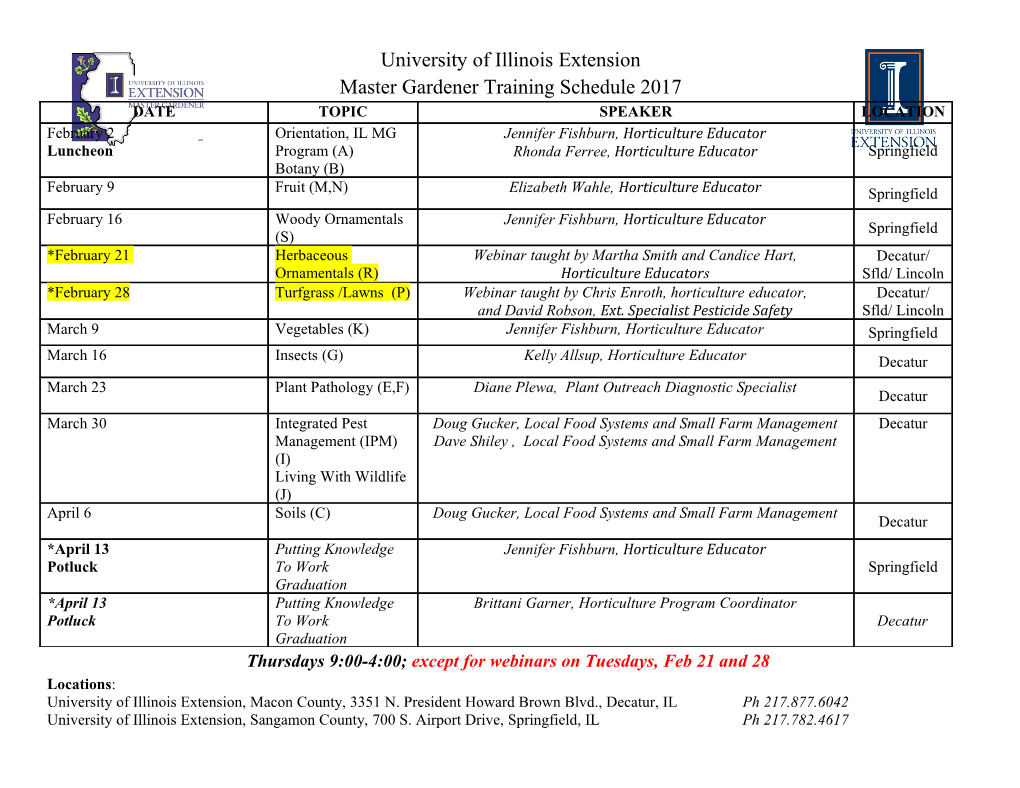
The anatomy of synapses – structural basis of long-term potentiation and synaptic scaling Kristen M. Harris Center for Learning and Memory, Section of Neurobiology, University of Texas at Austin, USA [email protected] This talk will emphasize work dedicated to understanding the role of dendritic spines and synapse structure in learning and memory. Long-term potentiation (LTP) of synaptic efficacy is a model of learning and memory that is well-suited to investigate this process. A clear understanding requires the nanometer resolution of three-dimensional (3D) reconstruction from serial section electron microscopy (ssEM). In this talk, I will review our work with ssEM to discover structural synaptic scaling, where synaptic input redistributes from many small to fewer enlarged spines with enlarged synapses by 2 hr after the induction of LTP. These enlarged spines sequester more core structures, including polyribosomes (involved in local protein synthesis), endosomes (a local source of plasma membrane, channels, and other signaling molecules), and smooth endoplasmic reticulum (an organelle that regulates calcium and receptor trafficking). Remarkably, the summed synaptic surface area per unit length of dendrite remains constant across control and LTP conditions. These findings support the hypothesis that competition for intrinsic dendritic resources regulates the number and size of synapses that a mature dendrite can support. I will also present new findings that show several presynaptic changes occur by 2 hr after the induction of LTP including a parallel loss of small axonal boutons and small dendritic spines; a homogeneous redistribution of presynaptic vesicles among the enlarged synapses; and a decrease in the number of presynaptic vesicles per synapse, suggesting either more presynaptic release or less recycling accompanies the expression of LTP. These findings support a synchrony between pre and postsynaptic elements that coordinates and refines synaptic circuitry to express LTP. Short biography Kristen M. Harris, PhD was born in Fargo, ND, and raised in the twin city of Moorhead, MN – her father, while born American, was of 100% Norwegian heritage from the Bergen area. She earned her BS, summa cum laude in Biology, Chemistry and Math, from the Minnesota State University Moorhead, her MS in Neurobiology at the University of Illinois with William Greenough, and her PhD at Northeastern Ohio Universities College of Medicine with Timothy Teyler – himself a postdoctoral graduate from Per Andersen’s laboratory at the University of Oslo. Harris had the great honor to be a member of an International Committee to examine 6 Ph.D. candidates from Prof. Per Andersen’s laboratory in 1995 (not the least of whom were the Mosers now at Trondheim). Hence, her Norwegian roots are strong. She went on to postdoctoral research at Harvard Medical School and remained there on the faculty for 15 years before moving to Boston University where she co-directed a Program in Neuroscience with Howard Eichenbaum. After 4 years at BU, she was recruited as a Georgia Research Alliance Eminent Scholar to the Medical College of Georgia where she built and directed the Synapses and Cognitive Neuroscience Center for 4 years, before moving to the University of Texas-Austin where she is now Professor and Fellow in the new Center for Learning and Memory. She served on the search committee to recruit Laura Colgin (a postdoctoral fellow from the Moser’s laboratory) to UT. She is the recipient of many awards including the National Institutes of Health Javits Merit Award. Her research is at the forefront of the systematic study of synapse structure, especially as it relates to the maturation of synaptic function and long-term potentiation. Spinal motor networks – excitation moving us forward Ole Kiehn Mammalian Locomotor Laboratory, Department of Neuroscience, Karolinska Institutet, Stockholm, Sweden [email protected] Brain functions are generated by activity in dedicated neural circuits. A major challenge to modern neuroscientist is to understand the function and mode of operation of such circuits in the complex mammalian brain. For locomotor behaviors, like walking, motor circuits in the spinal cord itself generate the actual timing and coordination of the rhythmic muscle activity. Excitatory neurotransmission is known to play a critical role in the generation of the locomotor rhythm and the drive in these spinal motor networks. Here, I will present data on the identification of excitatory networks in the mammalian spinal cord and brainstem locomotor networks. Among several classes of excitatory interneurons in the spinal cord, V2a interneurons are marked selectively by the expression of the transcription factor Chx10. Using combined physiological and molecular approaches we have determined the role of V2a interneurons in the locomotor network and shown that the V2a interneurons play little or no role in rhythm-generation but drive commissural interneurons to insure left-right alternation during locomotion. The V2a network is organized in a modular fashion along the cord and using genetic tracing and ablation and we show that the V2a interneurons together with other excitatory neurons control specific molecularly defined groups of commissural interneurons involved in left-right alternation. Using a transgenic mouse line that selectively drive light-sensitive channels in glutamatergic excitatory neurons in the spinal cord and brainstem we show that such neurons in the cord are directly responsible for rhythm- generation and that glutamatergic neurons in the lower hindbrain serve as command neurons for initiating locomotor activity. Surprisingly motor neurons may also - in addition to their classical transmitter acetylcholine - release glutamate from central synapses and activate locomotor networks. Together our experiments provide insights to the principal mode of operation of a large-scale mammalian motor circuit. Short biography Ole Kiehn is Professor at the Department of Neuroscience, Karolinska Institutet, Stockholm, Sweden. He obtained a medical degree in 1985 from University of Copenhagen where he also gained his doctoral degree in neurophysiology in 1990. Kiehn trained as a post-dotoctoral fellow at Cornell University in 1989- 90 where after he returned to University of Copenhagen to established his lab. In 2001 he was recruited to Karolinska Institutet. Kiehn’s work on motor neurons has provided new insights to the role of intrinsic membrane properties to normal motor function as well as their contribution to dysfunctional motor symptoms after spinal cord injury. His work on spinal locomotor circuitries controlling walking in mammals has defined some of the fundamental building blocks of the network, including interneuron circuitries controlling left-right coordination and inhibitory and excitatory interneuron networks engaged in pattern formation. In his work he has combined genetic manipulation with electrophysiological and anatomical techniques needed to understand complex network function in the mammalian brain. Kiehn is on the editorial board for Current Opinion of Neurobiology, Journal of Neurophysiology, and Neural Development among others. His is a member of the Nobel Assembly and his work has been acknowledged by the Schellenberg Prize and an endowed Söderberg’s professorship. Worms reveal how the brain works – functional genomics in C. elegans Cori Bargmann Howard Hughes Medical Institute, The Rockefeller University, New York, USA [email protected] All animals and even unicellular organisms recognize other members of their species and interact with them, suggesting that ancient biological systems are involved in these recognition processes. Innate social behaviors emerge from neuronal circuits that interpret sensory information based on an individual’s own genotype, sex, and experience. Our work centers on genes that regulate social behaviors of C. elegans, a nematode worm with a simple and well-characterized nervous system. A specific neuropeptide receptor has different activity levels in wild-type social strains and solitary strains of C. elegans. Neuropeptides like vasopressin and oxytocin and their receptors are also implicated in mammalian social behaviors, suggesting a common genetic vocabulary for sociality. How do genes and the enviroment interact to generate behavior? In C. elegans, a specialized “social brain” integrates the genetic regulation of social preference with environmental context. The genetic and anatomical hub of the social brain is a neuron called RMG, which connects to many other neurons through gap junction synapses. Sensory input into RMG determines whether the environment is appropriate or inappropriate for aggregation. If the environmental context is correct, RMG alters the animal’s behavioral preferences so that it approaches pheromones released by its companions. Regulation of RMG by the neuropeptide receptor switches animals between social and solitary behavioral modes. Short biography Cornelia Isabella Bargmann is Investigator, Howard Hughes Medical Institute, and Torsten N. Wiesel Professor and Head, Laboratory of Neural Circuits and Behavior, The Rockefeller University, New York. Education and Training. 1977-1981: B.S. with a major in Biochemistry, The University of Georgia, Athens, GA. Undergraduate research with Sidney Kushner. 1981-1987: Ph.D., Massachusetts Institute of Technology, Department of Biology. Doctoral research with Robert A. Weinberg at the MIT Cancer Center and the Whitehead Institute
Details
-
File Typepdf
-
Upload Time-
-
Content LanguagesEnglish
-
Upload UserAnonymous/Not logged-in
-
File Pages7 Page
-
File Size-