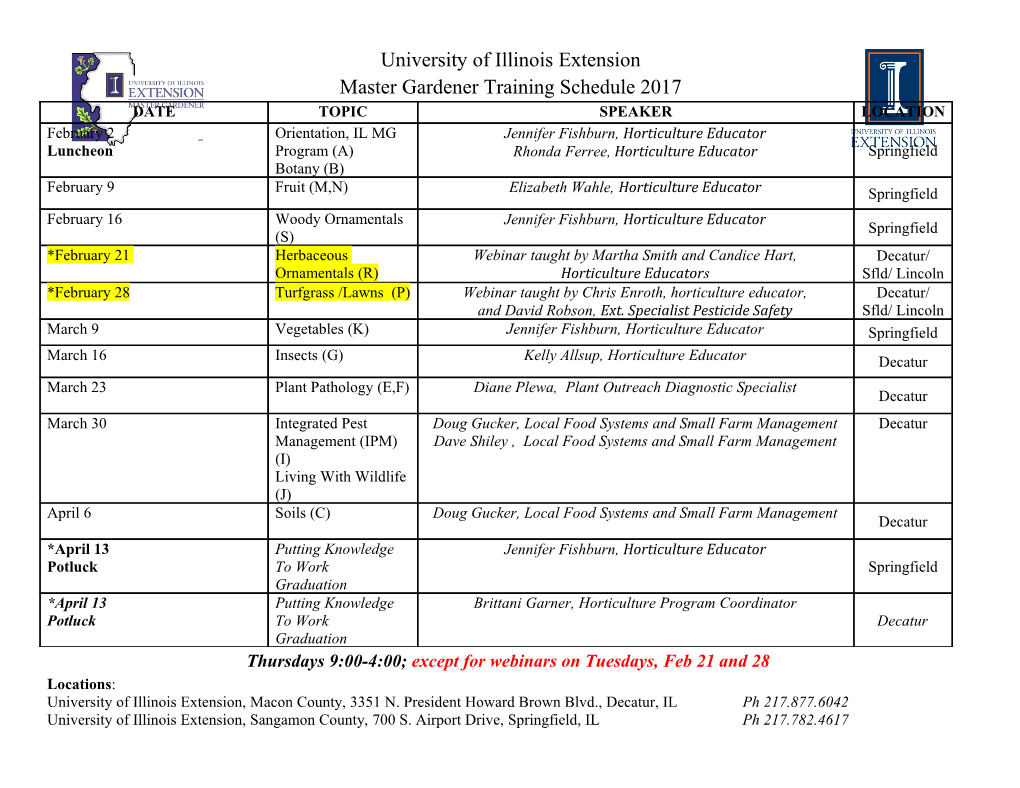
PRAMANA c Indian Academy of Sciences Vol. 86, No. 4 — journal of April 2016 physics pp. 819–836 Cluster decay of 112−122Ba isotopes from ground state and as an excited compound system K P SANTHOSH∗, P V SUBHA and B PRIYANKA School of Pure and Applied Physics, Kannur University, Swami Anandatheertha Campus, Payyanur 670 327, India ∗Corresponding author. E-mail: [email protected] MS received 28 March 2014; revised 20 February 2015; accepted 11 March 2015 DOI: 10.1007/s12043-015-1084-7; ePublication: 23 September 2015 Abstract. The decay properties of various even–even isotopes of barium in the range 112 ≤ A ≤ 122 is studied by modifying the Coulomb and proximity potential model for both the ground and excited state decays, using recent mass tables. Most of the values predicted for ground state decays 30 are within the experimental limit for measurements (T1/2 < 10 s). The minimum T1/2 value refers to doubly magic or nearly doubly magic Sn (Z = 50) as the daughter nuclei. A comparison of log10(T1/2) value reveals that the exotic cluster decay process slows down due to the presence of excess neutrons in the parent nuclei. The half-lives are also computed using the Universal formula for cluster decay (UNIV) of Poenaru et al and the Universal decay law (UDL) of Qi et al,andare compared with CPPM values and found to be in good agreement. A comparison of half-life for ground and excited systems reveals that probability of decay increases with a rise in temperature or otherwise, inclusion of excitation energy decreases the T1/2 values. Keywords. Cluster decay; alpha decay. PACS Nos 23.70.+j; 23.60.+e 1. Introduction Cluster radioactivity is an exotic natural radioactivity in which a particle heavier than 4He, but lighter than a fission fragment, is emitted. An important feature of this phenomenon is that decay products formed are in ground or the lowest excited state that contradicts the normal hot fission in which highly excited fragments are produced. This rare, cold phe- nomenon which forms an intermediate phenomenon between α decay and spontaneous fis- sion was first theoretically predicted by Sandulescu et al [1] in 1980 based on the quantum mechanical fragmentation theory (QMFT). The fact that cluster emission is marked by several alpha emissions is the reason for the rare nature of this process. In 1984, Rose and Jones [2] experimentally observed this phenomenon in the radioactive decay of 223Ra by emitting 14C. Much efforts have been done on both experimental and theoretical fronts Pramana – J. Phys., Vol. 86, No. 4, April 2016 819 K P Santhosh, P V Subha and B Priyanka for understanding the physics of cluster radioactivity. The next important step occurred in cluster studies is the observation of 24Ne from 231Pa by Tretyakova et al [3] in Dubna using solid-state nuclear track detectors (SSNTD). Intense experimental research led to the detection of about 20 cases of spontaneous emission from trans-lead nuclei with partial half-lives ranging from 1011 to 1028 s [4] emitting clusters ranging from 14Cto34Si. The common factor of all cluster radioactivity events is that the heavy-mass residue is found to be in the neighbourhood of the doubly-magic nucleus 208Pb. This makes cluster radioactivity to be characterized as ‘lead radioactivity’ and indicates strong influence of shell effects on the nature of this phenomenon. On the basis of analytical superasymmet- ric fission model (ASAFM) [5] and preformed cluster model (PCM) [6], a second island of heavy-cluster radioactivity was predicted in the decays of some neutron-deficient rare- earth nuclei into 100Sn (Z = N = 50) daughter or a neighbouring nucleus. Furthermore, another doubly closed 132Sn (Z = 50, N = 82) daughter radioactivity was predicted by Kumar et al [6] for decays of some selective neutron-rich rare-earth nuclei. Experimen- tally, several unsuccessful attempts [7–10] have been made to measure the radioactivity of 100Sn from the parent nucleus 116Ba in 58Ni + 58Ni reaction. Spontaneous fission is also a competing decay channel in some heavy cluster emitters [11]. The theoretical models that are used to explain cluster radioactivity are classified as fission-like and alpha-like models. In fission-like model [12], the nucleus deforms contin- uously as it penetrates the nuclear barrier and reaches scission configuration after running down the Coulomb barrier. In alpha-like model [13–17], the cluster is assumed to be pre- formed in the parent nuclei before it penetrates the nuclear interacting barrier. Recently, Ni et al [18] proposed a unified formula of half-lives for α decay and cluster radio- activity to study the decay of even–even nuclei. Ren et al [19,20] analysed cluster radioac- tivity using microscopic density-dependent cluster model with the renormalized M3Y nucleon–nucleon interaction and reproduced cluster decay half-lives using a new formula. In low-energy heavy-ion reactions, both the light (A ≈ 60) and medium mass (A ≈ 110) compound systems emit intermediate mass fragments (IMFs) with mass lighter than A ≈ 20 and they arise as multiple clusters and are accompanied by multiple light particles (Z ≤ 2). Light compound nuclei (CN) with mass number ACN < 44 that are formed in low-energy (E/A< 15 MeV/nucleon) heavy-ion reactions are highly excited and carry large angular momenta. It was found that the decay process must depend on temperature- and angular momentum-dependent potential barriers. Cluster decay of N = Z, A = 4n nucleus via α nuclei (A2 = 4n, Z2 = N2) clusters was first pointed out in 1988 by Gupta and collaborators [21]. For very light nuclei with mass number A ≤ 80 it was seen that the minima in potential energy surfaces lies always and only at α nuclei. Other clus- ters observed in the decay of radioactive nuclei also start appearing and become equally predominant for N/Z 1 parents, as the N/Z ratio becomes larger than one. As an extension of the phenomenon of exotic cluster radioactivity from various radioactive parents, the 12C emission from 112−120Ba nuclei has been subjected to many theoretical [22–25] and experimental [26,27] investigations. A new phenomenon of emis- sion of IMFs also referred to as ‘clusters’ or ‘complex fragments’ from excited compound 58 58 116 system was observed in Ni + Ni → Ba* reactions at both high (Ecm = 315 MeV) [28,29] and medium (Ecm = 174, 185.5, 187.5 and 197 MeV) energies [30,31]. The decay of Ba isotopes was first experimentally observed by Tretyakova et al [32] at Dubna and then by Guglielmetti et al [26] at GSI, Darmstadt. In all the experiments, 114Ba 820 Pramana – J. Phys., Vol. 86, No. 4, April 2016 Cluster decay of 112−122Ba isotopes isotope (Z = 56) was produced using on-line mass separators by 58Ni (58Ni, 2n) reaction. In Dubna experiments, polycarbonate track detectors and in GSI experiments phosphate glass detectors were used for searching carbon clusters. The experimental logarithm of half-life for 12C decay from 112Ba was found to be 3.75 s and that from 114Ba was 4.23 s. These results however could not be reproduced in experiments [9] carried out in GSI in 1997, and hence the above half-life values are taken as the experimental lower limit. According to La Commara et al [31], this decay was assumed to be from the excited compound systems. As the half-life values fall within the experimental upper limits for measurements, 4He, 8Be, 12C, 16O and 20Ne are predicted to be the possible decay modes of 112−120Ba. In the present paper, we have studied the decay of various even–even Ba isotopes 112−122Ba by modifying the Coulomb and proximity potential model (CPPM) proposed by Santhosh et al [33] in 2000. We would like to mention that, using CPPM, the authors had performed the ground-state cluster decays of 116−124Ce isotopes [34] and 107−116Xe isotopes [35], which revealed the role of doubly magic 100Sn daughter in cluster decay processes. The authors further modified CPPM by incorporating excitation energy and thus studied the cluster emission in superdeformed Sr isotopes formed in heavy-ion reac- tion [36]. The Coulomb and proximity potential model (CPPM) has been used extensively over the past decade for studying cluster/heavy particle decay [37–41], α-decay [42,43] and heavy-ion fusion [44]. In the present work, we have studied the decay of even–even 112−122Ba isotopes, by modifying the Coulomb and proximity potential model (CPPM), by incorporating the excitation energy and also by using the temperature-dependent radius, Ri and temperature-dependent surface diffuseness width, b. The present study looks into all aspects of α and cluster decay from these isotopes from both ground and excited states beginning with the identification of the most probable clusters from all isotopes. The decay properties have been studied and the results have been analysed to reveal the role of neutron and proton shell closure. The half-lives of these isotopes are computed and are compared with those values calculated using Universal formula for cluster decay (UNIV) of Poenaru et al [45] and Universal decay law (UDL) [46]. A plot of logarithmic half-life against fragment mass number is drawn for both the ground and the excited states decay. The Coulomb and proximity potential model (CPPM) is presented in detail in §2, the results and discussion of the study are given in §3 and a conclusion of the entire work is given in §4. 2. The Coulomb and proximity potential model (CPPM) The potential energy barrier, in the Coulomb and proximity potential model (CPPM), is taken as the sum of Coulomb potential, proximity potential and centrifugal potential for the touching configuration and for the separated fragments.
Details
-
File Typepdf
-
Upload Time-
-
Content LanguagesEnglish
-
Upload UserAnonymous/Not logged-in
-
File Pages18 Page
-
File Size-