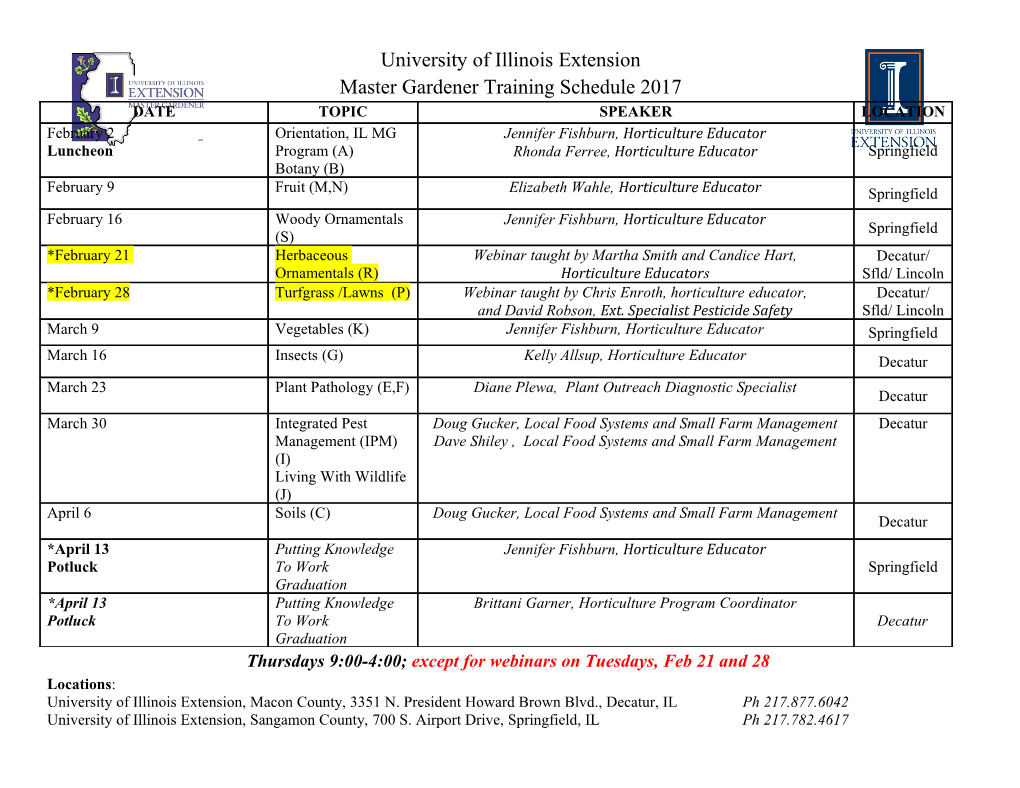
UNIVERSITY OF CALIFORNIA SANTA CRUZ HYBRID OPTOFLUIDIC BIOSENSORS A dissertation submitted in partial satisfaction of the requirements for the degree of DOCTOR OF PHILOSOPHY in ELECTRICAL ENGINEERING by Joshua W. Parks December 2016 The Dissertation of Joshua W. Parks is approved: Professor Holger Schmidt, Chair Professor Joel Kubby Professor Clair Gu Tyrus Miller Vice Provost and Dean of Graduate Studies Copyright © by Joshua W. Parks 2016 All rights reserved Table of Contents 1 Introduction ............................................................................................................ 1 2 Background ............................................................................................................ 5 2.1 Fluorophores and Fluorescence ....................................................................... 5 2.2 Fluorescent Reporters: Nucleic Acid Stains and Molecular Beacons ............. 8 2.3 ARROW Fundamentals ................................................................................. 12 2.3.1 Deriving the ARROW Condition ............................................................ 13 2.3.2 ARROW Device Fabrication .................................................................. 16 2.3.3 Fluorescence Based ARROW Detection ................................................ 20 2.4 PDMS Prototyping and Fabrication .............................................................. 24 3 Enhancement of ARROW Optofluidic Biosensors via Thermal Annealing ........ 29 3.1 Post Processing Low Temperature Thermal Annealing ................................ 32 3.2 Optical Characterization of Annealed ARROW Structures .......................... 32 3.2.1 Optical Confinement and Loss in Solid-core Waveguides ..................... 32 3.2.2 Transmission Changes in Connected Solid- and Liquid-core Waveguides 36 3.2.3 Repeatability of Waveguide Improvements ............................................ 39 3.3 Optofluidic Sensor Chip Improvement Upon Thermal Annealing ............... 41 4 Passive Hybrid Optofluidic Integration ................................................................ 44 4.1 Device Fabrication and Hybrid Integration ................................................... 46 4.2 Flow Properties of Hybrid Optofluidic Systems ........................................... 51 iii 4.3 On-Chip Mixing, Labelling and Detection of Single DNA ........................... 52 4.4 Microfluidic Sample Distribution to Multiple ARROW Waveguides .......... 57 4.4.1 Positive Pressure Driven Flow in Hybrid Systems ................................. 59 4.4.2 Passive Control of Fluid Velocity in Hybrid Systems ............................ 61 4.5 Microparticle Filtering and Distribution ........................................................ 62 5 Active Hybrid Optofluidic Integration ................................................................. 67 5.1 Microfluidic Automaton Fabrication and Control ......................................... 68 5.2 Integration of Microfluidic and Optofluidic Layers ...................................... 71 5.3 On-chip Fluid Control and Mixing Using an Active Hybrid Optofludiic System ..................................................................................................................... 72 5.4 Detailed Control Analysis of the Hybrid System .......................................... 74 5.5 Automated Single Nucleic Acid Detection ................................................... 74 5.6 Automated Nucleic Acid Isolation and Detection ......................................... 77 6 Detection of Clinically Relevant Biomolecules ................................................... 82 6.1 Optofluidic analysis system for amplification-free, direct detection of Ebola infection ................................................................................................................... 83 6.1.1 Systematic design of a hybrid optofluidic system for detection of single clinical nucleic acids ............................................................................................ 83 6.1.2 Automated pre-concentration of Ebola nucleic acids ............................. 85 6.1.3 Automated and specific detection of single, clinical ebola nucleic acids 87 6.2 MMI Based Wavelength Division Multiplexing for Optofluidic Detection of Single Virus Detection ............................................................................................. 91 iv 6.2.1 Multimode Interference Waveguides (MMIs) ........................................ 92 6.2.2 Optofluidic MMI Biosensor Architecture ............................................... 96 6.2.3 Virus sample preparation ........................................................................ 98 6.2.4 Multiplex virus fluorescence detection setup and experiment ................ 99 6.2.5 Multiplexed single virus detection with single color labeling .............. 100 6.2.6 Multiplexed single virus detection with combinatorial labeling ........... 103 6.2.7 WDM Biosensing Analysis Considerations .......................................... 107 7 Flexible PDMS optofluidic waveguide platform with multi-dimensional reconfigurability and functionality ........................................................................... 113 7.1 Fabrication of multilayer flexible optofluidic platforms ............................. 114 7.2 Loss in PDMS Waveguides ......................................................................... 116 7.3 Comparison of PDMS and ARROW Detection Chips ................................ 122 7.5 PDMS Optofluidic Waveguide Chips with Multi-dimensional Reconfigurability ................................................................................................... 127 7.5.1 Dynamically Reconfigureable PDMS MMI Waveguide ...................... 128 7.5.2 Lightvalve Architecture for Simultaneous Control of Fluid and Light 131 7.5.3 Lightvavle Trap with Single Bioparticle Analysis Capability .............. 136 8 Conclusions and Outlook ................................................................................... 139 9 Appendixes ......................................................................................................... 141 10 Bibliography ................................................................................................... 182 v List of Figures Fig. 2.1 | Organic dye properties: Cy3 and Cy5 dyes as examples. ........................ 6 Fig. 2.2 | Binding modes of nucleic acid stains. ........................................................ 8 Fig. 2.3 | YO and YOYO intercalating dye properties. ........................................... 9 Fig. 2.4 | Molecular Beacons for Nucleic Acid Detection. ...................................... 11 Fig. 2.5 | Generic ARROW Structure and Wavevector Diagram. ....................... 13 Fig. 2.6 | Fabrication workflow for solid- and liquid-core ARROWs. (Dimensions not precisely to scale) ........................................................................................ 17 Fig. 2.7 | ARROW Detection Geometry. a ARROW fluorescence 3D view with butt coupled excitation and collection optical fibers. b Cross-sectional view of the excitation volume with an antibody labeled virus being excited. ....................... 20 Fig. 2.8 | Fluorescence Detection on an ARROW Chip. a Bulk fluorescence detection of 22.5 nM Cy5 dye. b Detection of single fluorescent 100 nm 7 Fluospheres (488 nm excitation, cfluorsphere = 4 x 10 particles/mL). ................... 21 Fig. 2.9 | Common geometries of pneumatically addressable PDMS microvalves. The push-down valve is shown end-on while the membrane and lifting gate valves are shown from the side for clarity. ......................................................... 25 Fig. 2.10 | General multilayer PDMS device fabrication workflow. .................... 27 Fig. 3.1 | Reduction of tantalum oxide photoluminescence in thin films via thermal annealing. Note the red line indicates as-grown film photoluminescence. ........................................................................................... 29 vi Fig. 3.2 | Physical effects of thermal annealing on ARROW structures. a, Tantalum oxide thin film (100 nm) “star” cracking after 700 °C annealing. b, Cross-sectioned liquid- to solid-core waveguide intersection with a large crack caused by annealing. ***Secondary feature is an artifact of focused ion beam milling. ................................................................................................................ 30 Fig. 3.3 | Scanning electron microscope (SEM) image (left) and schematic representation (right) of a 4 µm wide solid-core ARROW ridge waveguide sitting on a silicon pedestal. .............................................................................. 31 Fig. 3.4 | Effects of annealing on solid-core waveguide light propagation. a, Top down image of light confinement within an ARROW ridge waveguide before (top) and after annealing (bottom). b, Integrated mode intensity output of a 1 cm long as-grown (top) and annealed (bottom) solid-core waveguide. Intensity within the core of the waveguide is shaded dark blue. c, Transmission measurements for cut-back solid core waveguides. As-grown waveguides (open -1 blue squares) have a fitted loss coefficient αAG = 1.63 cm (dashed line). Annealed waveguides (closed red circles) have a fitted loss coefficient αA = 0.471 cm-1 (solid line). Both fits have r2 > 0.99. ................................................. 33 Fig. 3.5 | Finite difference method simulations of a solid-core
Details
-
File Typepdf
-
Upload Time-
-
Content LanguagesEnglish
-
Upload UserAnonymous/Not logged-in
-
File Pages233 Page
-
File Size-