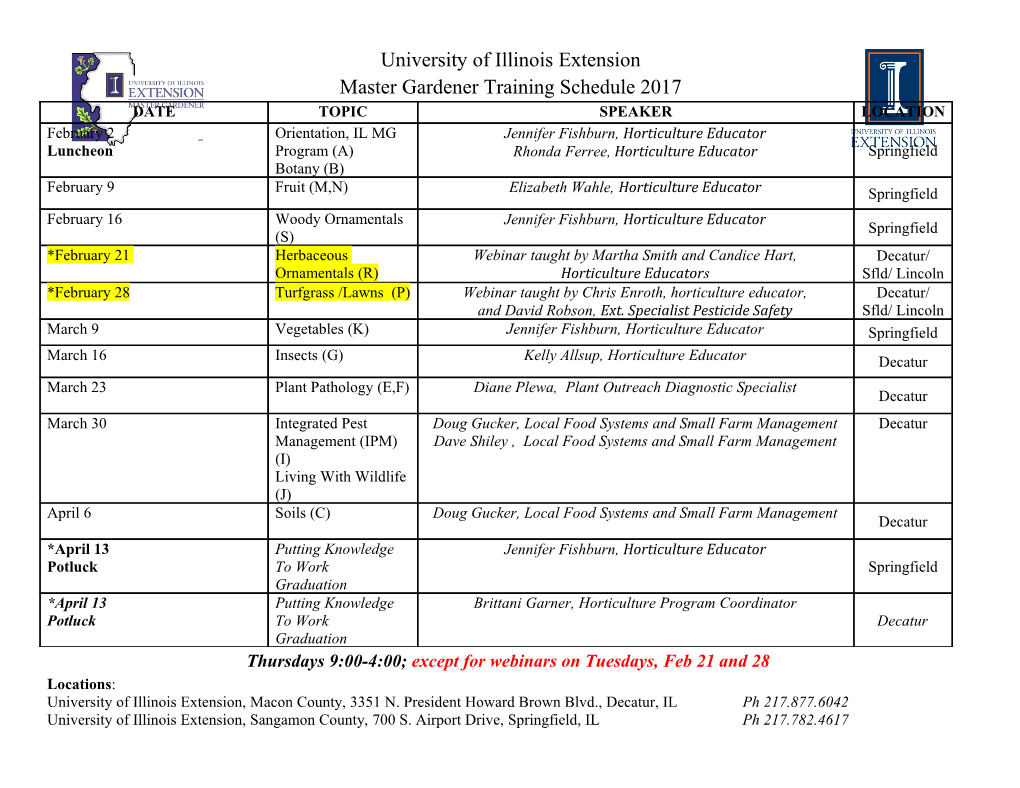
review article The relationship between liquid, supercooled and glassy water Osamu Mishima & H. Eugene Stanley 8 . ....................................................................................................................................................................................................................................................... That water can exist in two distinct `glassy' formsÐlow- and high-density amorphous iceÐmay provide the key to understanding some of the puzzling characteristics of cold and supercooled water, of which the glassy solids are more- viscous counterparts. Recent experimental and theoretical studies of both liquid and glassy water are now starting to offer the prospect of a coherent picture of the unusual properties of this ubiquitous substance. < 2 8 At least one `mysterious' property of liquid water seems to have been unreachable temperature Ts 45 C (refs 2, 5), at which point recognized 300 years ago1: whereas most liquids contract as tem- the entire concept of a `liquid state' becomes dif®cult to sustain. perature decreases, liquid water begins to expand when its tem- Water is a liquid, but glassy waterÐalso called amorphous iceÐ perature drops below 4 8C. A kitchen experiment demonstrates that can exist when the temperature drops below the glass transition the bottom layer of a glass of unstirred iced water remains at 4 8C temperature Tg (about 130 K at 1 bar). Although glassy water is a while colder layers `¯oat' on top; the temperature at the bottom rises solid, its structure exhibits a disordered liquid-like arrangement. only after all the ice has melted. Low-density amorphous ice (LDA) has been known to exist for The mysterious properties of liquid water become more pro- 60 years (ref. 6), and a second kind of amorphous ice, high-density nounced in the supercooled region below 0 8C (refs 2±4; Fig. 1). For amorphous ice (HDA), was discovered in 19847±9. Thus ice has example, if the coef®cient of thermal expansion, isothermal com- two different amorphous forms. This phenomenon is called pressibility, and constant-pressure speci®c heat are extrapolated polyamorphism, a term meaning that the pure material can exist below the lowest temperatures at which these properties are mea- in more than one amorphous state10,11. All amorphous solid forms - surable (about 38 8C at 1 bar) they appear to become in®nite at an of H2O can be partitioned into these two low-density and high- T (°C) T (K) Superheated water (metastable) 100 TB 373 60 333 46 Stable water 319 20 Classic models? 293 4 TMD Percolation picture? 277 0 T 273 M Supercooled water Stability-limit conjecture? (metastable) -35 238 -38 TH 235 -45 Ts 228 "No man's land" Liquid-liquid phase transition hypothesis? -120 TX 150 Ultraviscous water (metastable) -140 Tg 130 Glassy water Singularity-free scenario? (metastable) Figure 1 Schematic illustration of different temperature domains, at atmospheric functions. The region between the homogeneous nucleation curve TH(P) and the pressure, of H2O. One domain is stable; the others are metastable. Indicated on crystallization curve TX(P) is a kind of `no-man's-land', as experiments on the liquid the right side of the diagram are the approximate temperature domains which phase cannot be performed. The temperatures denoted 60 8C, 46 8C, 20 8C and proposed explanations are intended to describe. All the indicated numbers are 4 8C indicate the onset of anomalies in the sound velocity, isothermal 2,3 experimentally observed (and are de®ned in the text), except the number denoted compressibility, shear viscosity, and density, respectively . TMD denotes the Ts (about -45 8C) which is a ®tting parameter that emerges from assuming the temperature of maximum density, TB the boiling temperature, TM the melting existence of a power-law singularity in measured thermodynamic response temperature, and Tg the glass transition temperature. Nature © Macmillan Publishers Ltd 1998 NATURE | VOL 396 | 26 NOVEMBER 1998 | www.nature.com 329 review article density forms, although there may be slight variations in the liquid, Whalley et al.36 predicted from thermodynamic arguments individual structures depending on the preparation method and the location of the low-temperature metastable melting line of ice 12±15 13 procedure . IhÐa prediction later con®rmed by experiments Ðwhich located LDA was originally produced by depositing water vapour onto a the ice Ih melting line above the supposed glass transition tempera- 6 cold plate or by cooling micrometre-sized droplets of liquid ture Tg. Below Tg, amorphization does not occur on the extrapola- water extremely rapidly12,16,17. It was once believed that LDA could tion of the two-phase melting line, as once believed37, but rather be nanocrystalline because of its structural resemblance to the melting is `delayed' to higher pressures13 as suggested in ref. 36 most common form of ice, ice Ih (ref. 18). Now it is agreed that (Fig. 3). This delayed melting can be interpreted as a shift from two- LDA is amorphous, as it transforms to a highly viscous liquid19 when phase melting to one-phase melting. heated to the known glass transition temperature of about 130 K Here we discuss the status of experimental knowledge of liquid 8 (refs 20±22). and glassy water in the light of recent theoretical attempts to obtain In contrast, HDA was discovered by compressing ice Ih below a a uni®ed picture of the liquid, supercooled and glassy states and the temperature of about 150 K (refs 7, 13, 23). HDAwas independently transformations between them. These theoretical proposals typi- 8,24 discovered by bombarding an ice-Ih layer with an electron beam . cally focus on the thermodynamic anomalies exhibited by super- The transformation from a crystalline phase to a (presumably cooled water, offering explanations for the singularity-like metastable) amorphous phase is called amorphization25,26 (Fig. 2). behaviour that seems to occur at about -45 8C. However, it has When compressed further at low enough temperatures, HDA proved very dif®cult to ®nd discriminating experimental tests that crystallizes and becomes a high-density crystalline ice23,27. will allow the theoretical models to be evaluated, and the matter is In addition to the original preparation methods from the vapour, still unresolved. Computer simulations are unable to resolve the liquid or crystalline phases of water, LDA can be formed directly issue either, because water is notoriously hard to simulate realistically from HDAÐand vice versa (Fig. 2). This `polyamorphic transition' and the results can be highly sensitive to the intermolecular is accompanied by a surprisingly sharp and surprisingly large potential functions used. Nonetheless, we hope to show that the volume changeÐmore than 20%Ðwhen thermodynamic param- picture is now becoming clearer, in that some experiments do seem eters such as temperature or pressure change in®nitesimally. The to lend support to speci®c theoretical models, while simulations properties of the amorphous ices change apparently dis- have elucidated interrelationships between them. In particular, we continuously at the polyamorphic transition. For example, the can start to discern the origins of water's anomalous behaviour shear modulus of LDA decreases on compression, jumps discon- within the nature of its intermolecular forces. The prospect of a tinuously at the LDA ! HDA transition, and then increases with uni®ed picture of water now seems to be in view. further compression28. Therefore, the transformation between LDA and HDA appears to be a ®rst-order transition between two Hypotheses different metastable amorphous `phases', rather than a relaxation Many classic `explanations' for the mysterious behaviour of liquid phenomenon in which an unstable amorphous structure changes water have been developed38±42, including a simple two-state model continuously from LDA to HDA. Simulations also reveal such dating back to RoÈntgen43 and a clathrate model dating back to polyamorphic transitions27,29. Pauling44. Here we shall brie¯y describe three relatively recent HDA has a structure similar to that of high-pressure liquid water, hypotheses under active current discussion. suggesting that HDA is a glassy form of high-pressure water30±32. One of the popular explanations is the stability limit hypothesis45, Thus far, however, the glass transition of HDA has not been observed. which assumes that the spinodal temperature line Ts(P) in the There is a continuing discussion about whether pressure-induced pressure±temperature (P±T) phase diagram connects at negative P amorphization13,26,33,34 is conventional `two-phase melting' (crystal- to the locus of the liquid-to-gas spinodal (the limit of metastability) line solid becoming liquid) at a temperature below Tg, or is what is for superheated water (Fig. 4a). It is assumed that liquid water called `one-phase melting' (a collapse transitionÐsolid becoming cannot exist when cooled or stretched beyond the line Ts(P). 35 collapsed solid) (Fig. 3). Thus it remains unresolved whether one The data supporting the singularity at Ts are limited to tempera- considers HDA to be a glassy state of liquid water or to be a collapsed tures several degrees above Ts. Hence it is possible that functions `ill-crystalline' phase. Regarding HDA as a glass of a high-pressure have a rapid rise but that there is no singularity, behaviour predicted boiling polyamorphic T G L L condensation transition eva 2-phase p melting 1-phase o rati melting rel sub on lting (collapse) ion on a t li x a me mati atio ns ificati e r dep n on d zing t HDL free osition vi con Tg polymorphic crystallization polyamorphic ice-Ih HDA X X A A transition amorphization transition exp. Figure 2 Transitions between gas (G), liquid (L), crystal (X) and amorphous (A) phases of water. We note that the amorphous `phase' is metastable and non- ergodic (meaning a non-equilibrium state in a metastable phase), so the three P transitions to and from `A' are different from the other transitions because `A' has many local minima; thus these transitions are not true thermodynamic transitions, Figure 3 Two-phase melting contrasted with one-phase melting.
Details
-
File Typepdf
-
Upload Time-
-
Content LanguagesEnglish
-
Upload UserAnonymous/Not logged-in
-
File Pages7 Page
-
File Size-