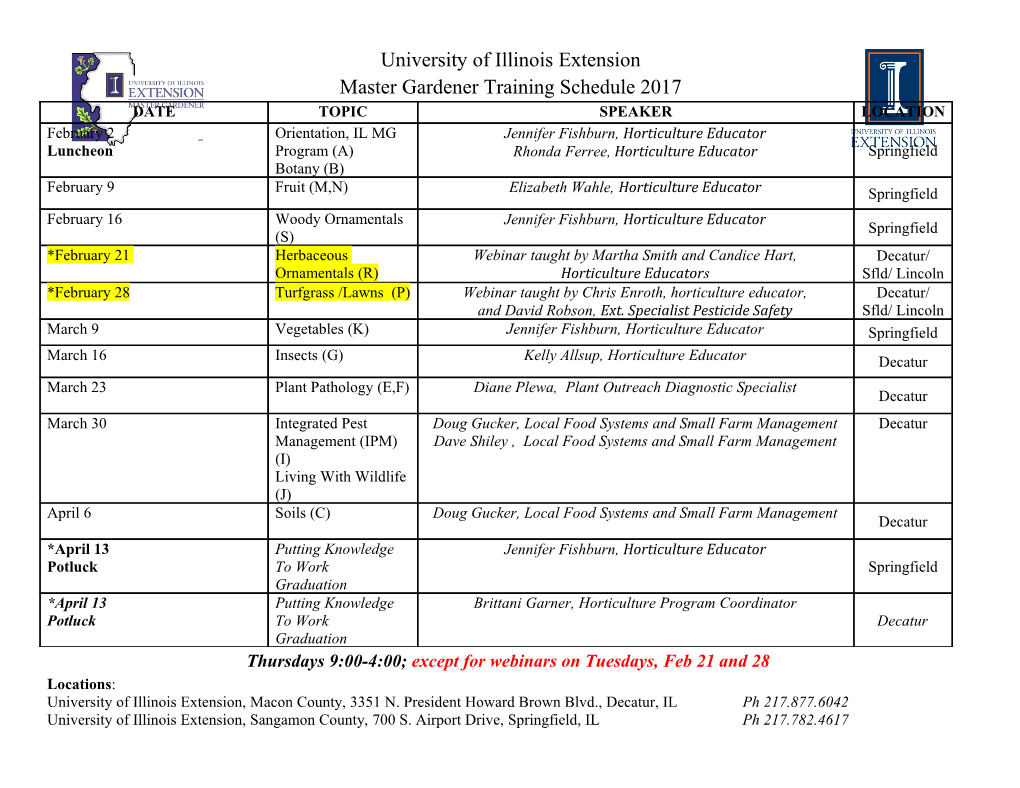
The role of BAG6 in protein quality control A thesis submitted to The University of Manchester for the degree of Doctor of Philosophy in the Faculty of Biology, Medicine and Health 2017 Yee Hui Koay School of Biological Sciences List of contents Page List of tables 5 List of figures 6 List of abbreviations 9 Abstract 13 Declaration 14 Copyright statement 14 Acknowledgement 15 Chapter 1: Introduction 1.1 Protein folding and quality control 16 1.2 Degradation of misfolded proteins 21 1.3 Protein biosynthesis and quality control at the endoplasmic reticulum 24 1.4 BAG6 31 1.4.1 BAG6 structure 32 1.4.2 BAG6 in tail-anchored protein targeting 34 1.4.3 BAG6 in degradation of mislocalised proteins 35 1.4.4 BAG6 triages targeting and degradation 39 1.4.5 BAG6 in endoplasmic reticulum-associated degradation 43 1.5 Aims and objectives of study 46 Chapter 2: Materials and methods 2.1 DH5α competent cells preparation 47 2.2 Plasmid DNA preparation 47 2.3 Plasmid construction 49 2.3.1 Myc-BirA-pcDNA5/FRT/TO 49 2.3.2 BAG6-myc-BirA-pcDNA5/FRT/TO 51 2.3.3 BAG6(∆N)-myc-BirA-pcDNA5/FRT/TO 51 2.3.4 HA3-XBP1u G519C(∆HR2)-pcDNA3.1(+) 52 2.4 Cell culture 52 2 2.5 Stable inducible cell line generation and induction 53 2.6 siRNA transfection 53 2.7 Transient transfection for immunoblotting and immunofluorescence microscopy 54 2.8 Treatment with proteasome and lysosomal protease inhibitors 55 2.9 Endo Hf treatment 55 2.10 Cycloheximide chase 55 2.11 SDS-PAGE and immunoblotting 56 2.12 Immunofluorescence microscopy 58 2.13 Cell cracking 59 2.14 Co-immunoprecipitation 60 2.15 Denaturing immunoprecipitation 60 2.16 BioID 61 2.17 Bioinformatics 62 2.18 Data and statistical analysis 62 Chapter 3: Role of BAG6 and UBR4 in ERAD 3.1 Introduction 64 3.2 BAG6 contributes to degradation of Op91 66 3.3 BAG6 and UBR4 are involved in ERAD of opsin-degron 71 3.4 UBR4 does not associate stably with BAG6 or opsin-degron 73 3.5 Depletion of UBR4 increases opsin-degron ubiquitination 75 3.6 UBR4 knockdown increases association of BAG6 with 80 opsin-degron and RNF126 3.7 Discussion 82 Chapter 4: Novel role of BAG6 in the UPR 4.1 Introduction 87 4.2 BAG6 interacts with XBP1u 90 4.3 BAG6 is required for efficient XBP1u degradation 95 4.4 BAG6 interacts with the C-terminal hydrophobic region of full length XBP1u in order to promote degradation 99 4.5 BAG6 depletion impairs XBP1u ubiquitination 103 3 4.6 Discussion 105 Chapter 5: The use of BioID to identify potential BAG6 substrates and/or interacting factors 5.1 Introduction 111 5.2 Generation and characterisation of BAG6-myc-BirA construct 113 5.3 Optimisation of BioID protocol 117 5.4 The use of transient expression system for efficient biotinylation 120 5.5 Mass spectrometry data analysis 123 5.5.1 Comparison of technical replicates 123 5.5.2 Pre-enrichment analysis of myc-BirA vs BAG6-myc-BirA 125 5.5.3 Comparison of biological replicates 126 5.5.4 Identification of potential BAG6 substrates 128 5.5.5 Identification of potential BAG6 interacting factors 135 5.6 Discussion 138 Chapter 6: Conclusions and future work 6.1 Conclusions 146 6.2 Future work 148 Chapter 7: References 150 Chapter 8: Supplementary data 165 Final word count: 41748 4 List of tables Page Table 2.1 Plasmid constructs used in this study 48 Table 2.2 Standard reaction setup and PCR cycling condition for site-directed mutagenesis using KOD Hot Start DNA Polymerase 50 Table 2.3 PCR cycling condition for subcloning 51 Table 2.4 siRNA sequences used in this study 54 Table 2.5 Primary and secondary antibodies used in immunoblotting 57 Table 2.6 Primary and secondary antibodies used in immunofluorescence microscopy 59 Table 5.1 Mean LFQ intensity of proteins found in both biological replicates and having at least two times higher intensity with BAG6-myc-BirA sample than myc-BirA sample 131 Table 5.2 Hydrophobicity prediction using Kyte and Doolittle scale with window size of 20 and threshold of 0 136 Table 5.3 Potential BAG6-associated protein quality control factors with gene ontology related to ubiquitination pathway 139 5 List of figures Page Figure 1.1 Illustration on a protein folding energy landscape 18 Figure 1.2 The Hsp70 and TRiC/CCT chaperone systems 20 Figure 1.3 The ubiquitination cascade and chain formation 22 Figure 1.4 The three major protein translocation pathways: co- or post-translational translocation dependent on Sec61 and Sec61-independent post-translational translocation 25 Figure 1.5 The mammalian endoplasmic reticulum-associated degradation 28 Figure 1.6 The mammalian unfolded protein response signalling pathways 30 Figure 1.7 Schematic representation of human BAG6 protein domains and its putative binding partners 33 Figure 1.8 The yeast and mammalian guided entry of tail-anchor pathway components 36 Figure 1.9 Model illustrating BAG6 role in protein quality control in the cytosol 37 Figure 1.10 Model illustrating BAG6 role in endoplasmic reticulum pre-emptive quality control 40 Figure 1.11 Current model illustrating BAG6 triages protein targeting and degradation 41 Figure 1.12 Model illustrating BAG6 role in endoplasmic reticulum- associated degradation 44 Figure 3.1 UBR family proteins and the mammalian N-end rule pathway 65 Figure 3.2 BAG6 overexpression stabilises Op91 and causes nuclear re-localisation of Op91 67 Figure 3.3 Degradation of glycosylated form of Op91 is delayed with BAG6 knockdown 70 Figure 3.4 Degradation of opsin-degron is delayed with BAG6 and UBR4 knockdown 72 Figure 3.5 BAG6 and opsin-degron do not co-immunoprecipitate UBR4 74 Figure 3.6 UBR4 knockdown does not significantly alter BAG6 steady state level 76 6 Figure 3.7 UBR4 knockdown increases total cellular ubiquitination and BAG6-associated polyubiquitinated species 78 Figure 3.8 UBR4 knockdown increases opsin-degron ubiquitination 79 Figure 3.9 UBR4 knockdown increases opsin-degron-BAG6 and BAG6- RNF126 interactions 81 Figure 3.10 Proposed model on UBR4 function in ERAD in correlation with BAG6 86 Figure 4.1(A) Schematic representation of human XBP1 protein domains 88 Figure 4.1(B) Model illustrating the splicing event of XBP1 mRNA under ER stress 88 Figure 4.2 BAG6 re-localises and stabilises XBP1u 91 Figure 4.3 BAG6 co-purifies XBP1u with proteasome inhibition 94 Figure 4.4 Proteasome inhibition delays XBP1u degradation 96 Figure 4.5 BAG6 knockdown delays XBP1u degradation 98 Figure 4.6 UBR4 and RNF126 knockdown have no effect on XBP1u degradation 99 Figure 4.7 BAG6 cannot interact with XBP1 variant lacking HR2. XBP1 HR2 mutant is more stable and does not require BAG6 for degradation 101 Figure 4.8 BAG6 knockdown decreases XBP1u ubiquitination 104 Figure 4.9 Proposed model on BAG6-mediated XBP1u degradation 108 Figure 5.1 Model for application of BioID method 112 Figure 5.2 BAG6-myc-BirA re-localises and stabilises Op91 114 Figure 5.3 RIPA buffer extracts more proteins and fewer proteins are being washed away 118 Figure 5.4 Transient overexpression system is used for BioID experiment 121 Figure 5.5 Box plots of LFQ intensity and MS/MS count with respect to the three experiment conditions and technical replicates for BAG6-myc-BirA and BAG6-myc-BirA+Bz samples 124 Figure 5.6 Venn diagrams, histograms and scatter plots of proteins identified from LFQ intensity and MS/MS count of myc-BirA and BAG6-myc-BirA samples 127 7 Figure 5.7(A) Box plot of non-normalised and median-normalised LFQ intensities with respect to the four experiment conditions and two biological replicates except for Bag6(∆N)-myc-BirA with no biological replicate 129 Figure 5.7(B) Venn diagram of proteins identified from myc-BirA sample of two biological replicates 129 Figure 5.7(C) Venn diagram of proteins identified from BAG6-myc-BirA sample of two biological replicates 129 Figure 5.7(D) Dendrogram showing how the experiments cluster 129 Figure 5.8 Flow chart for the identification of potential BAG6 substrates 130 Figure 5.9 Venn diagram of proteins identified from BAG6-myc-BirA and BAG6(∆N)-myc-BirA samples 137 8 List of abbreviations ADP adenosine diphosphate AMP adenosine monophosphate AP-MS affinity purification coupled to mass spectrometry ATE1 arginyl-tRNA--protein transferase 1 ATF4 cyclic AMP-dependent transcription factor ATF-4 ATF6 cyclic AMP-dependent transcription factor ATF-6 ATP adenosine triphosphate BAG6 BCL2-associated athanogene 6 BAT3 HLA-B associated transcript 3 BiP immunoglobulin heavy chain-binding protein BirA biotin ligase CAML calcium-modulating cyclophilin ligand CHIP E3 ubiquitin-protein ligase CHIP DMEM Dulbecco’s Modified Eagle’s Medium EDEM1 ER degradation-enhancing alpha-mannosidase-like protein 1 eIF2α eukaryotic translation initiation factor 2-alpha Endo Hf endoglycosidase Hf ER endoplasmic reticulum ERAD endoplasmic reticulum-associated degradation ER pQC endoplasmic reticulum pre-emptive quality control GET guided entry of tail-anchor gp78 E3 ubiquitin-protein ligase AMFR HECT homologous to the E6-AP carboxyl terminus Hrd1 E3 ubiquitin-protein ligase synoviolin Hsc70 heat shock cognate 71 kDa protein Hsp70 heat shock 70 kDa protein Hsp40 heat shock 40 kDa protein 9 IPOD insoluble protein deposit IRE1 serine/threonine-protein kinase/endoribonuclease IRE1 JUNQ juxta nuclear quality control compartment KCMF1 E3 ubiquitin-protein ligase KCMF1 LC-MS/MS liquid chromatography-tandem mass spectrometry LC3 microtubule-associated proteins 1A/1B light chain 3 LFQ label-free quantification MARCH6 E3 ubiquitin-protein ligase MARCH6 NLS nuclear localisation signal NO nitric oxide Npl4 nuclear protein localization protein 4 homolog NTAN1 protein N-terminal asparagine
Details
-
File Typepdf
-
Upload Time-
-
Content LanguagesEnglish
-
Upload UserAnonymous/Not logged-in
-
File Pages178 Page
-
File Size-