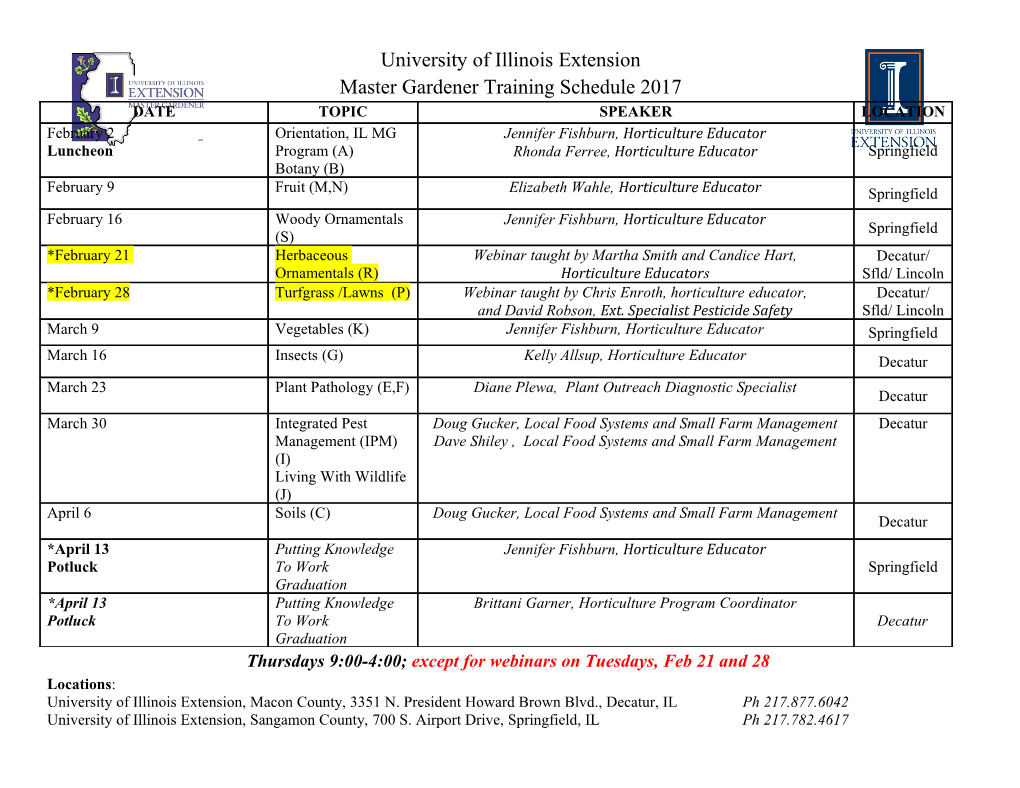
01 02 03 Chapter 3 04 05 06 07 08 Gene Regulation During Cold Stress Acclimation in Plants 09 10 11 Viswanathan Chinnusamy, Jian-Kang Zhu, and Ramanjulu Sunkar 12 13 14 15 Abstract 16 17 Cold stress adversely affects plant growth and development and thus limits crop productivity. Diverse 18 plant species tolerate cold stress to a varying degree, which depends on reprogramming gene expres- 19 sion to modify their physiology, metabolism, and growth. Cold signal in plants is transmitted 20 to activate CBF-dependent (C-repeat/drought-responsive element binding factor-dependent) and 21 CBF-independent transcriptional pathway, of which CBF-dependent pathway activates CBF regulon. CBF transcription factor genes are induced by the constitutively expressed ICE1 (inducer of CBF 22 expression 1) by binding to the CBF promoter. ICE1–CBF cold response pathway is conserved in diverse 23 plant species. Transgenic analysis in different plant species revealed that cold tolerance can be significantly 24 enhanced by genetic engineering CBF pathway. Posttranscriptional regulation at pre-mRNA processing 25 and export from nucleus plays a role in cold acclimation. Small noncoding RNAs, namely micro-RNAs 26 (miRNAs) and small interfering RNAs (siRNAs), are emerging as key players of posttranscriptional gene 27 silencing. Cold stress-regulated miRNAs have been identified in Arabidopsis and rice. In this chapter, 28 recent advances on cold stress signaling and tolerance are highlighted. 29 Key words: Cold stress, second messengers, CBF regulon, CBF-independent regulation, ICE1, 30 posttranscriptional gene regulation. 31 32 33 34 35 1. Introduction 36 37 38 Temperature profoundly influences the metabolism of organisms 39 and thus is a key factor determining the growing season and 40 geographical distribution of plants. Cold stress can be classi- 41 fied as chilling (<20◦C) and freezing (<0◦C) stress. Temperate 42 plants have evolved a repertoire of adaptive mechanisms such as 43 seed and bud dormancy, photoperiod sensitivity, vernalization, 44 45 46 R. Sunkar (ed.), Plant Stress Tolerance, Methods in Molecular Biology 639, DOI 10.1007/978-1-60761-702-0_3, © Springer Science+Business Media, LLC 2010 47 48 39 40 Chinnusamy, Zhu, and Sunkar 49 supercooling (prevention of ice formation in xylem parenchyma ◦ 50 cells up to homogenous ice nucleation temperature, −40 C), and 51 cold acclimation. In cold acclimation, plants acquire freezing tol- 52 erance on prior exposure to suboptimal, low, nonfreezing tem- 53 peratures. The molecular basis of cold acclimation and acquired 54 freezing tolerance in Arabidopsis and winter cereals has been stud- 55 ied extensively. Plants modify their metabolism and growth to 56 adapt to cold stress by reprogramming gene expression during 57 cold acclimation (1, 2). This chapter briefly covers cold stress sig- 58 naling, transcriptional and posttranscriptional regulation of gene 59 expression in cold acclimation process, and the genetic engineer- 60 ing of crops with enhanced cold tolerance. 61 62 63 64 65 2. Cold Stress 66 Sensing 67 68 Thus far, the identity of stress sensor in plants is unknown. The 69 fluid mosaic physical state of the plasma membrane is vital for 70 the structure and function of cells, as well as to sense tempera- 71 ture stress. The plasma membrane undergoes phase transitions, 72 from a liquid crystalline to a rigid gel phase at low temperature 73 and to a fluid state at high temperature. Thus, a decrease in tem- 74 perature can rapidly induce membrane rigidity at microdomains. 75 Further, protein folding is influenced by temperature changes. 76 Temperature-induced changes in the physical state of mem- 77 branes and proteins are expected to change the metabolic reac- 78 tions and thus the metabolite concentrations. Therefore, plant 79 cells can sense cold stress through membrane rigidification, pro- 80 tein/nucleic acid conformation, and/or metabolite concentration 81 (a specific metabolite or redox status). 82 In alfalfa and Brassica napus, cold stress-induced plasma 83 membrane rigidification leads to actin cytoskeletal rearrangement, 2+ 2+ 84 induction of Ca channels, and increased cytosolic Ca level. 85 These events induce the expression of cold-responsive (COR) 86 genes and cold acclimation. Further, a membrane rigidifier ◦ 87 (DMSO) can induce COR genes even at 25 C, whereas a mem- 88 brane fluidizer (benzyl alcohol) prevents COR gene expression ◦ 89 even at 0 C (3, 4). Genetic evidence for plants sensing cold stress 90 through membrane rigidification is from the study of the fad2 91 mutant impaired in the oleic acid desaturase gene of Arabidop- 92 sis. In wild-type Arabidopsis plants, diacylglycerol (DAG) kinase ◦ 93 is induced at 14 C. The fad2 mutant (more saturated mem- 94 brane) and transgenic Arabidopsis overexpressing linoleate desat- ◦ 95 urase gene showed the expression of DAG kinase at 18 and 12 C, 96 respectively (5). Cold Tolerance in Plants 41 97 98 3. Second 99 Messengers 100 and Signaling 2+ 101 Cytosolic Ca levels act as second messenger of the cold stress 102 signal (6). Calcium may be imported into the cell or released from 103 intracellular calcium stores. Patch-clamp studies of cold-induced 104 potential changes of the plasma membrane in Arabidopsis mes- 105 ophyll protoplasts showed the cold-activatedcalcium-permeable 2+ 106 channel involved in the regulation of cytosolic Ca signatures 2+ 107 (7). Membrane rigidification induced cytosolic Ca signatures; 108 and COR gene expression was impaired by gadolinium, a 2+ 109 mechanosensitive Ca channel blocker, which suggests the 2+ 110 involvement of mechanosensitive Ca channels in cold acclima- 111 tion (4). Pharmocological studies implicated cyclic ADP-ribose- 112 and inositol-1,4,5-triphosphate (IP3)-activated intracellular 113 calcium channels in COR gene expression (4). Calcium influx 114 into the cell appears to activate phospholipase C (PLC) and D 115 (PLD), which produce IP3 and phosphatidic acid, respectively. 2+ 116 IP3 can further amplify Ca signatures by activation of IP3-gated 117 calcium channels (8). Genetic analysis revealed that loss-of- 118 function mutants of FIERY1 (FRY1) inositol polyphosphate 119 1-phosphatase show significantly higher and sustained levels 120 of IP3 instead of the transient increase observed in wild-type 121 plants. This situation leads to higher induction of COR genes 122 and CBFs, the upstream transcription factors (9). In addition, 123 the calcium exchanger 1 (cax1)mutantofArabidopsis, which is 2+ + 124 defective in a vacuolar Ca /H antiporter, exhibited enhanced 125 expression of C-repeat binding factor/dehydration responsive 126 element binding (CBF/DREB) proteins and their target COR 2+ 127 genes (10). Therefore, cytosolic Ca signatures are upstream of 128 the expression of CBFs and COR genes in cold stress signaling. 129 Cold acclimation induces accumulation of ROS such as 130 H2O2, both in chilling-tolerant Arabidopsis and chilling-sensitive 131 maize plants. ROS can act as a signaling molecule to reprogram 2+ 132 transcriptome probably through induction of Ca signatures and 133 activation of mitogen-activated protein kinases (MAPKs) (11) 134 and redox-responsive transcription factors. Arabidopsis frostbite1 135 (fro1) mutant, which is defective in the mitochondrial Fe-S sub- 136 unit of complex I (NADH dehydrogenase) of the electron trans- 137 fer chain, shows a constitutively high accumulation of ROS. 138 This high accumulation of ROS in fro1 results in reduced COR 139 gene expression and hypersensitivity to freezing stress, probably 140 because of desensitization of cells by the constitutively high ROS 141 expression (12). 142 Cold stress-induced second messenger signatures can be 143 decoded by different pathways. Calcium signatures are sensed 144 by calcium sensor family proteins, namely calcium-dependent 42 Chinnusamy, Zhu, and Sunkar 145 protein kinases (CDPKs), calmodulins (CaMs), and salt overly 146 sensitive 3-like (SOS3-like) or calcineurin B-like (CBL) pro- 147 teins. In a transient expression system in maize leaf protoplast, a 148 constitutively active form of an Arabidopsis CDPK (AtCDPK1) 149 activated the expression of barley HVA1 ABA-responsive pro- 150 moter::LUC reporter gene suggesting that AtCDPK is a posi- 151 tive regulator in stress-induced gene transcription (13). Genetic 152 and transgenic analyses implicated CDPKs as positive regulators, 153 but a calmodulin, a SOS3-like or a CBL calcium binding protein, 154 and a protein phosphatase 2C (AtPP2CA) are negative regulators 155 of gene expression and cold tolerance in plants. Components of 156 MAPK cascades are induced or activated by cold and other abi- 157 otic stresses. Genetic and transgenic analyses showed that MAPKs 158 act as a converging point in abiotic stress signaling. ROS accu- 159 mulation under these stresses might be sensed through a MAPK 160 cascade (14). ROS activates the AtMEKK1/ANP1 (MAPKKK)– 161 AtMKK2 (MAPKK)–AtMPK4/6 (MAPK) MAPK cascade, which 162 positively regulates cold acclimation in plants (11). Many of these 163 phosphorylated proteins show activation or induction of gene 164 expression under multiple stress conditions, and genetic modi- 165 fication results in alteration of multiple stress responses. These 166 results suggest that the proteins act as connecting nodes of stress 167 signal networks. Identification of the target proteins or transcrip- 168 tion factors of protein kinase or phosphatase cascades will shed 169 further light on stress signaling. 170 171 4. Transcriptional 172 Regulation 173 174 Chilling-tolerant plants reprogram their transcriptome in 175 response to acclimation temperature. Cold-regulated genes con- 176 stitute about 4–20% of the genome in Arabidopsis (15). The 177 promoter region of many COR genes of Arabidopsis con- 178 tains C-repeat (CRT)/DREs, initially identified in the promoter 179 of responsive to dehydration 29A (RD29A/COR78/LTI78). 180 As well, ABA-responsive elements are present in many cold- 181 induced genes. Genetic screens using dehydration and cold stress- 182 responsive promoter-driven LUCIFERASE (RD29A::LUC and 183 CBF3::LUC) led to the isolation of mutants, which unraveled 184 cold-responsive transcriptional networks.
Details
-
File Typepdf
-
Upload Time-
-
Content LanguagesEnglish
-
Upload UserAnonymous/Not logged-in
-
File Pages17 Page
-
File Size-