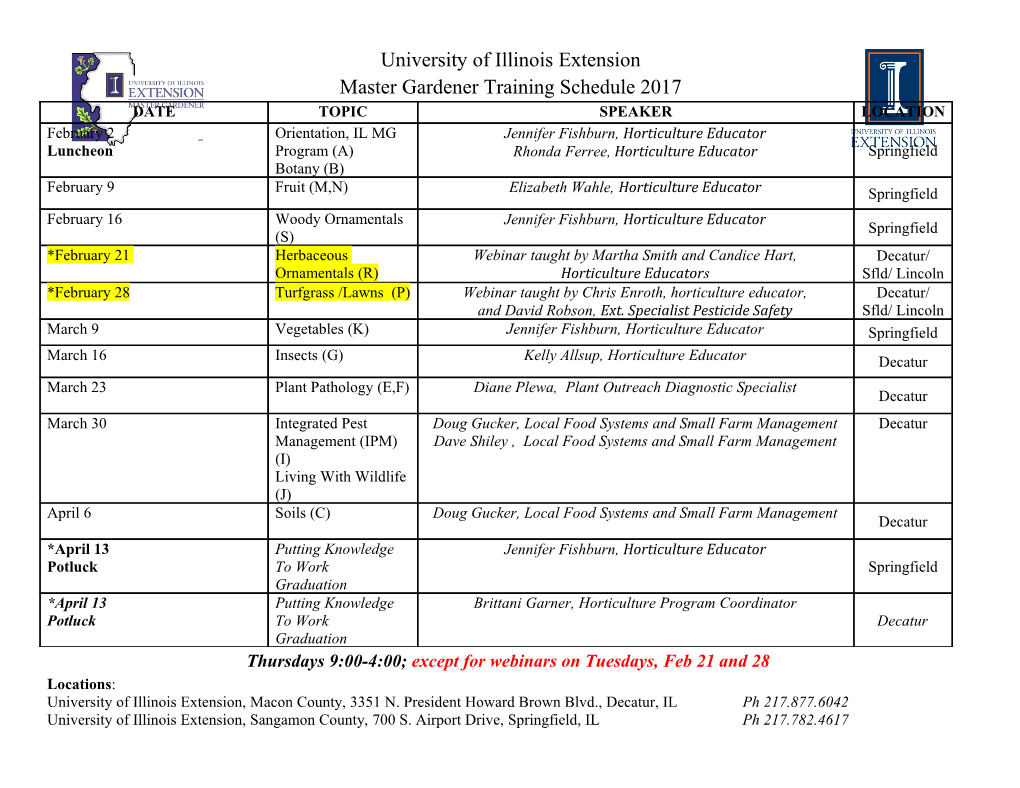
Research Article Cite This: ACS Sustainable Chem. Eng. 2019, 7, 19631−19639 pubs.acs.org/journal/ascecg α‑ Electrochemical CO2 Fixation to Methylbenzyl Bromide in Divided Cells with Nonsacrificial Anodes and Aqueous Anolytes Jury J. Medvedev, Xenia V. Medvedeva, Feng Li, Thomas A. Zienchuk, and Anna Klinkova* Department of Chemistry, University of Waterloo, 200 University Avenue West, Waterloo, Ontario N2L 3G1, Canada *S Supporting Information ABSTRACT: Electrocarboxylation of organic halides repre- sents a CO2 utilization strategy and a green alternative for the synthesis of many industrially relevant carboxylic acids. However, current electrocarboxylation methods rely on the utilization of sacrificial metal anodes, which are not sustainable, require high voltages, and complicate the understanding of the reaction mechanism. Here, we demonstrate the feasibility of performing electrocarboxylation reactions in divided cells with aqueous anolytes and nonsacrificial anodes, thereby eliminat- ing the reliance on sacrificial anodes and opening the door for coupling of this important reduction process with various electrooxidation reactions requiring aqueous electrolytes. Specifically, we report a detailed study of electrocarboxylation of (1-bromoethyl)benzene at a silver cathode coupled with an oxygen evolution reaction at a platinum anode in a divided cell with organic and aqueous compartments separated by ion-exchange membranes of different types. We examine how operating parameters, including membrane type, applied potential, substrate concentration, electrolyte, and temperature affect the overall process and the reaction product distribution. Based on the extensive experimental results, we propose a detailed mechanism for major electrochemical product formation accounting for both aprotic and protic environments. Systematic analysis and mechanistic insights presented in this study are expected to enable a rational catalyst, electrolyte, and system design tailored to fi ff electroorganic CO2 xation with di erent organic substrates to obtain industrially relevant carboxylic acids at practical potentials and currents. KEYWORDS: CO2 utilization, electrocarboxylation, electroorganic synthesis, cell design, membrane, reaction mechanism ■ INTRODUCTION products in EC reactions also causes electrode passivation, resulting in large current fluctuations (potentiostatic electrol- As climate change continues to accelerate, novel technologies 15 that reduce or use excess greenhouse gas emission will play a ysis) or increase in the cell voltage (constant current electrolysis).16 In addition, cathode passivation hinders the Downloaded via UNIV OF WATERLOO on February 2, 2020 at 19:35:03 (UTC). vital role in mitigating the negative impact of human activity 15 fi on our environment. One approach is to develop catalytic completion of the reaction. Replacing sacri cial anodes with alternative anodic reactions at nonsacrificial anodes could offer technologies that convert CO2 into value-added chemicals See https://pubs.acs.org/sharingguidelines for options on how to legitimately share published articles. using renewable electricity.1 Besides direct CO electro- a potential solution to overcome these limitations. While 2 divided cells with nonsacrificial anodes have been reported for reduction to single- and multicarbon small molecules, CO2 17−20 can be electrochemically coupled to various precursors to EC of several organic substrates, for halides any mentions − 21,22 produce a plethora of carboxylic acids.2 5 are limited to unsuccessful attempts. Specifically, electrocarboxylation (EC) of organic halides Despite EC reactions of organic halides with Mg or Al (R−X) has attracted attention as a green alternative for the anodes giving high yields of carboxylation products, their exact synthesis of nonsteroidal anti-inflammatory drugs, such as formation mechanism remains unclear. Electrochemical − 6 reduction of R X in the presence of CO2 is a three-step ibuprofen, and important precursors in industrial processes, • • 7,8 3,4 process: (1) halide reduction to a radical R , (2) R reduction such as cyanoacetic acid, an, and many others. It has been − shown that EC of organohalides with different cathodes (e.g., to the corresponding anion R , and (3) nucleophilic addition − − 23 2 5 fi of R to CO2 with a formation of carboxylate anion. Ag, Cu, and Ni) in undivided cells with sacri cial Mg or Al 9−14 anodes generally results in the formation of corresponding Mechanistic interpretations in previous works operated on 2+ carboxylic acids with moderate to high yields in the form of the assumption that electrochemically produced Mg ions − magnesium (or aluminum) salts.9 14 Although the formation of these salts simplifies the work-up procedure, it negatively Received: August 13, 2019 affects the reaction atom economy due to the anode Revised: November 4, 2019 consumption in the course of reaction. Formation of insoluble Published: November 12, 2019 © 2019 American Chemical Society 19631 DOI: 10.1021/acssuschemeng.9b04647 ACS Sustainable Chem. Eng. 2019, 7, 19631−19639 ACS Sustainable Chemistry & Engineering Research Article participated in the reaction only by interacting with the anodes, we contemplated performing this reaction in a divided carboxylate anion to form a corresponding salt24 (Scheme 1, electrochemical cell with a well-established process at the anodic side. Direct CO electrolyzers typically rely on the pathway I). 2 − oxygen evolution reaction (OER) as an anodic process,35 37 Scheme 1. Possible Pathways of Organic Halide although other oxidation reactions are now considered as well Carboxylation in Undivided Electrochemical Cells with Mg to minimize the cell voltage for their commercial implementa- Sacrificial Anodes tion.35,38 The mechanism of OER and catalysts for this reaction are well-established due to their importance in water splitting for electrochemical hydrogen production.39,40 The difficulty of adopting OER for EC lies in poor solubility of organic substrates in aqueous electrolytes and the need of an aprotic environment for the organic substrate to avoid intermediate protonation.23,41 We hypothesized that this complication can be overcome by introducing an appropriate membrane that can render the aprotic and aqueous compart- ment segregation. Cation-exchange membranes (CEMs) have been previously used in EC reactions in fully organic electrolytes to provide counterions for the carboxylate anions generated at the cathode.3,17,20 However, released halide ions (Hal−) accumulate in the catholyte, causing unwanted side − However, R can also uptake Mg2+ ion to form a Grignard reactions (alkene formation due to the Hal−-catalyzed 25,26 3,42,43 reagent that selectively reacts with CO2, producing a elimination reaction). In addition, in the case of an 27 corresponding carboxylate with quantitative yields (Scheme aqueous anolyte and an aprotic catholyte, CEM may enable 1, pathway II). In fact, the formation of Grignard reagents from competing the hydrogen evolution reaction (HER) at the organic halides in electrochemical cells with Mg anodes has 25,26 cathode by allowing the passage of protons. An anion-exchange been observed in the absence of CO2. Moreover, the membrane (AEM), whose performance has never been studied majority of reported EC of R−X was performed at constant 2−5,9−14 in EC reactions, could address these issues; however, with this current conditions, resulting in inevitable large membrane, counterions for the carboxylate anions can only be potential fluctuations.16 Consequently, at high negative 2+ 0 ° − provided by the catholyte, resulting in its consumption. A potentials, the reduction of Mg to Mg [E = 2.38 V vs bipolar membrane (BPM), which consists of anion and cation- standard hydrogen electrode (SHE)] at the cathode could also exchange layers, combines the properties of both and facilitate the Grignard reagent formation28 (Scheme 1, pathway potentially may either solve or exacerbate the problems III), in addition to enabling competing CO2 electroreduc- 29 associated with CEM and AEM. tion. Other sacrificial anodes (e.g., Al and Zn) have similar reactivity: generated Zn2+ or Al3+ can be cathodically reduced Here, we report a detailed study of EC of organic halides in to their metal forms, which, in addition to causing cathode a divided cell with organic and aqueous compartments 16 separated by various ion-exchange membranes using (1- passivation, produce organometallic compounds by reacting − with organic halides.30,31 These organometallic compounds bromoethyl)benzene (R Br) as a model substrate molecule. can react with CO , producing corresponding carboxylic This new strategy for EC of organic halides does not require − 2 fi acids.32 34 sacri cial anodes, instead relaying on a stable Pt anode and Thus, the chemistry of R−X reduction in the presence of OER as an anodic process. We investigate how operating fi CO2 in undivided cells with sacri cial anodes is rather complex parameters (membrane type, applied potential, substrate and convoluted, with carboxylate anions potentially originating concentration, electrolyte, and temperature) affect the overall from the electrochemically assisted Grignard reagent, rather process and the reaction product distribution. Based on the than the direct EC process. extensive experimental results, we propose a detailed Aiming to assess the potential of purely electrochemical mechanism for major electrochemical products formation fi fi xation of organohalides to CO2 decoupled from sacri cial supported by density functional
Details
-
File Typepdf
-
Upload Time-
-
Content LanguagesEnglish
-
Upload UserAnonymous/Not logged-in
-
File Pages9 Page
-
File Size-