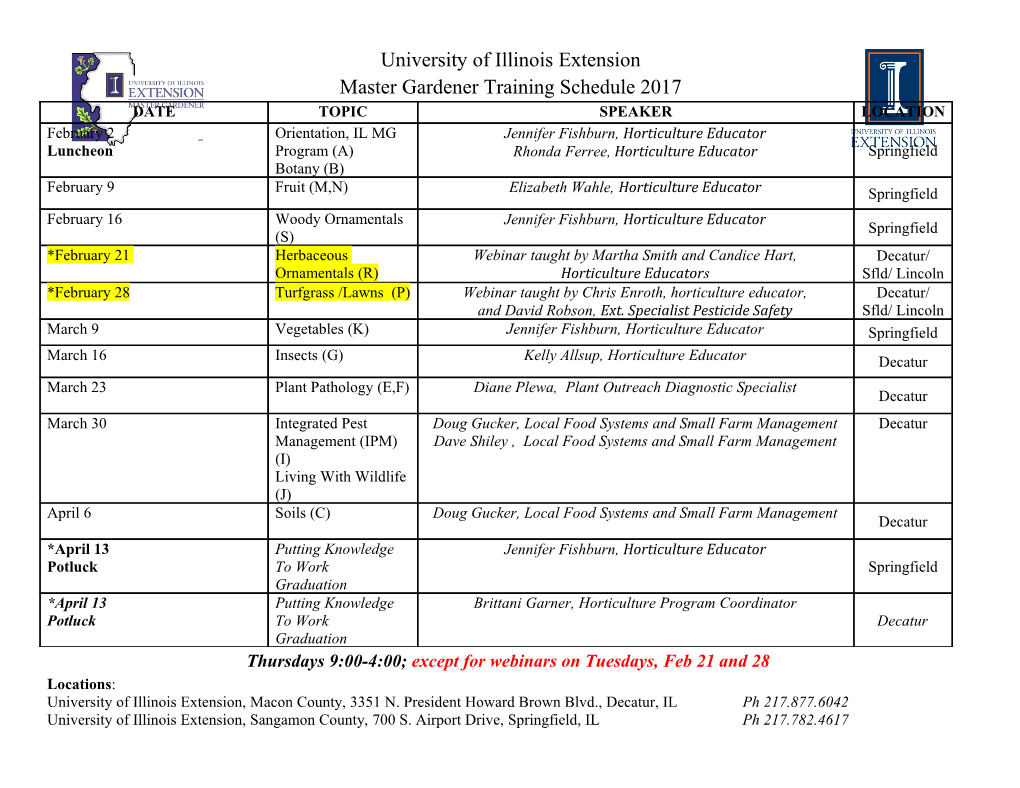
DEVELOPMENT OF SPINAL CIRCUITS FOR SWIMMING IN ZEBRAFISH (DANIO RERIO) LARVAE Emphasizing on the rhythm generation mechanism Yann Roussel Supervisor: Dr. Tuan Bui Examiners: Dr. Emily Standen, Dr. John Lewis and Dr. Michael Hildebrand External examiner: Dr. Joe Fetcho A thesis submitted in partial fulfillment of the requirements for the Doctorate in Philosophy degree in Biology Department of Biology Faculty of Science University of Ottawa © Yann Roussel, Ottawa, Canada, 2018 Table of content ABSTRACT viii RESUMÉ x PREFACE xii ACKNOWLEDGMENTS xiii Introduction 1 Motor control and the spinal cord 1 Locomotion 2 Swimming in zebrafish and its development 7 Neurogenesis in zebrafish larvae spinal cord 10 Changes in spinal circuitry during early development of zebrafish 11 Chapter 1: A Developmental Switch in the Operation of Larval Zebrafish Spinal Locomotor Circuits 17 ABSTRACT 18 Significance Statement 19 Introduction 20 Materials and Methods 23 Animal Care 23 Video recording and analysis 23 Animal preparation for electrophysiology 24 Chemogenetic ablation of DA neurons 24 Extracellular recordings 25 Intracellular recordings 26 Fluorescent imaging and cell counts 26 Data Analysis 27 Results 30 Increasingly stronger effect of strychnine on rhythmogenesis from 3 to 5 dpf 30 Differential effect of strychnine along the rostrocaudal axis 31 Secondary motoneurons preferentially generated caudally at 3 dpf 33 Arrhythmic synaptic inhibition to motoneurons becomes rhythmic at 3 dpf 33 Chemical ablation of dopaminergic neurons does not preclude a WGDR to SGDR transition 34 Modelling the maturation of zebrafish swimming with two coupled oscillators 36 Discussion 39 Proposed maturation of the neural control of swimming in developing zebrafish 40 Role of dopaminergic neurons in pattern generation 43 #ii Future directions 43 Chapter 2: Testing mechanisms of rhythmogenesis in spinal locomotor circuits of the developing zebrafish spinal cord 58! ABSTRACT 59 Introduction 60 Methods 63 Animal Care 63 Animal preparation for electrophysiology 63 Extracellular recordings 63 Intracellular recordings 64 ZAP 65 Data Analysis 65 Results 69 Lack of spinal neurons with subthreshold rhythmogenic intrinsic properties 69 Gap junctions are dispensable to the rhythmic tail beats after 3 dpf 71 Blocking persistent sodium current decreases the rhythm of tail beats in burst swimming fish 73 Dual patch-clamp recordings further confirm establishment of network oscillators at 5 dpf 74 Discussion 77 SUPPLEMENTARY DATA 88 Chapter 3: Modelling the maturation of swimming in Zebrafish (Danio rerio) through the development of spinal circuits 90! ABSTRACT 91 Introduction 92 Methods 96 Modelling environment 96 Modelling of single neurons 96 Modelling synapses 96 Spatial arrangement of spinal neurons 97 Noise in the network 98 Musculoskeletal model 99 Results 100 Part 1. Early locomotor behaviour, coiling, is encoded by a fully electrical network 100 Coiling results from unilateral gap-junction coupling 100 Network description 101 Simulation results 102 Part 2. Double coiling, an intermediate state toward swimming generated by a hybrid spinal circuit 103 iii# Double coiling is generated by a balance between excitatory and inhibitory commissural neurons 103 Network description 104 Simulation results 105 Part 3. First step of swimming: Burst swimming 106 Network oscillators drive episodes of tail beats 106 Network description 107 Simulation results 108 Part 4. Transitioning to beat-and-glide swimming 109 Network oscillators completely take over 109 Network description 110 Simulation results 112 Discussion 115 PM based network for early simple behaviour 115 Network oscillator for more refined movements 115 Competing during a transition period 117 Mechanisms of transition of burst to beat-and-glide swimming 118 Conclusion 138! Identification of mechanisms for rhythm generation in developing zebrafish larvae 138 Future directions 142 Switch in intrinsic properties 142 Identify sources of synaptic inhibition during swimming 142 Future maturation mechanism to integrate turns and slow/fast swimming modules 143 Incorporate sensory feedback in model 145 Incorporate supraspinal centers in model 146 Comparison with other animal models 147 Appendixes 150! Appendix 1 150 Ouabain does not perturbed rhythm generation nor episode duration 150 Discussion 151 Appendix 2 154 Pyridoxine-dependent epilepsy in zebrafish caused by aldh7a1 deficiency 154 Appendix 3 155 Insights into the genotypic/phenotypic spectrum and the pathophysiology of PLPBP-deficiency from novel B6-responsive clinical features, cellular, yeast and zebrafish models 155 Bibliography 156 iv# LIST OF TABLES Table 4.1. Parameter values of simple spiking neuron models of all populations involved in the modelling process 133! Table 4.2. Electrical synapse (gap junctions) weights used for modelling. 134! Table 4.3. Chemical synapses (glutamatergic in white and glycinergic in grey) weights used for modelling. Pre-synaptic neurons are in columns. Post-synaptic neurons in rows. 135! Table 4.4. Glutamatergic (white) and glycinergic (grey) reversal potential and time constants used to model respective synapses. 136 #v LIST OF FIGURES Figure 1.1. Schematic of the two general mechanisms for rhythm generation in the spinal cord. 14 Figure 1.2. Main types of spinal interneurons involved in motor control according to their morphological classification in the Zebrafish larva. 15 Figure 2.1. Larval zebrafish tail beat frequency during swimming is mainly between 20 and 40 Hz. 45 Figure 2.2. Greater effect of strychnine on tail beat rhythmicity at 5 dpf than at 3 and 4 dpf. 47 Figure 2.3. Effect of strychnine on fictive swimming in spinalized fish at 3 dpf and 5 dpf. 49 Figure 2.4. Differential effect of strychnine along the rostrocaudal axis of the zebrafish. 50 Figure 2.5. Cell count of secondary motoneurons and Chx10+ spinal neurons. 52 Figure 2.6. IPSCs mature from arrhythmic at 3 dpf to rhythmic with a frequency close to that of tail beats at 5 dpf during swimming episodes. 53 Figure 2.7. Metronidazole-treated Tg(dat:NTR-CFP) fish exhibit a development profile closer to 5 dpf rather than 3 dpf. 54 Figure 2.8. Coupled oscillator model of architectural change from pacemaker to network oscillator-based spinal locomotor circuits of developing zebrafish. 56 Figure 3.1. Subthreshold frequency preference analysis of caudal spinal neurons fails to identify pacemakers. 80 Figure 3.2. Carbenoxolone (CBX) reinforces the effect of strychnine at 3dpf but is dispensable at 4 and 5 dpf. 82 Figure 3.3. Riluzole disturbs rhythm of tail beats but only at 3 dpf. 84 Figure 3.4. Dual recordings in beat-and-glide swimming fish. 86 Figure 3.S1. Typical spiking activity of current-clamped spinal motoneurons (MN) and interneurons (IN). 88 Figure 3.S2. K-mean clustering of impedance profiles features on projection over PC1 and PC2. 89 Figure 4.1. Musculo-skeletal model helps to read network output. 123 Figure 4.2. Single coiling model relies on a fully electrical network driven by pacemaker neurons. 124 Figure 4.3. Double coiling model relies on a hybrid network of electrical and chemical synapses. 125 Figure 4.4. Emergence of half-centre oscillators during burst swimming. 127 Figure 4.5. Beat-and-glide swimming model requires both the implementation of a new population of neurons and maturation of intrinsic properties of existing neurons. 129 Figure 4.6. Beat-and-glide model network with adaptive CINs and MNs and tonic IIAs reproduces experimental observations of tail beat rhythm generation. 131 Video 4.S1 Single coiling model behavior 137 Video 4.S2 Double coiling model behavior 137 Video 4.S3 Burst swimming model behavior 137 Video 4.S4 Beat-and-glide swimming model behavior 137 Figure Appendix.1. Ouabain has little to no effect on tail beats rhythm or episode rhythm. 153 vi# LEGEND 5-HT : serotonin CPG : central pattern Mtz : metronidazole CaP : caudal primary generator PF : pattern formation CBX : carbenoxolone CoSA : commissural PM : pacemaker CEN : commissural secondary ascending pMN : primary motoneuron excitatory neuron DA : dopamine RG : rhythm generation CiA : circumferential Dpf : days-post-fertilization Rilu : riluzole ascending FFT : fast Fourier transform RoP : rostral primary CiD : circumferential Glu : glutamate/ SGDR : Strongly glycine descending glutamatergic dependent rhythm CIN : commissural Gly : glycine/ glycinergic sMN : secondary inhibitory neuron Hpf : hours-post-fertilization motoneuron CNS : central nervous IC : ipsilateral caudal Str : strychnine system IED : ipsilateral excitatory UCoD : unipolar CoBL : commissural descending commissural descending bifurcating longitudinal IIA : ipsilateral inhibitory VeLD : ventral longitudinal CoLA : commissural ascending descending! longitudinal ascending IN : interneuron WGDR : Weakly glycine CoLo : commissural local MCoD : multipolar dependent rhythm CoPA : commissural primary commissural descending ascending MiP : middle primary MN : motoneuron #vii ABSTRACT It has long been established that the spinal cord is able to produce locomotor activity on its own. Despite extensive research identifying and describing the involvement of multiple spinal neuron populations that are part of the spinal locomotor circuit, the manner in which these different components act together to precisely control the rhythm and the pattern of activation of muscles during locomotion remains largely undetermined. We sought to shed light on how the components of spinal locomotor circuits interact to produce robust locomotion
Details
-
File Typepdf
-
Upload Time-
-
Content LanguagesEnglish
-
Upload UserAnonymous/Not logged-in
-
File Pages285 Page
-
File Size-