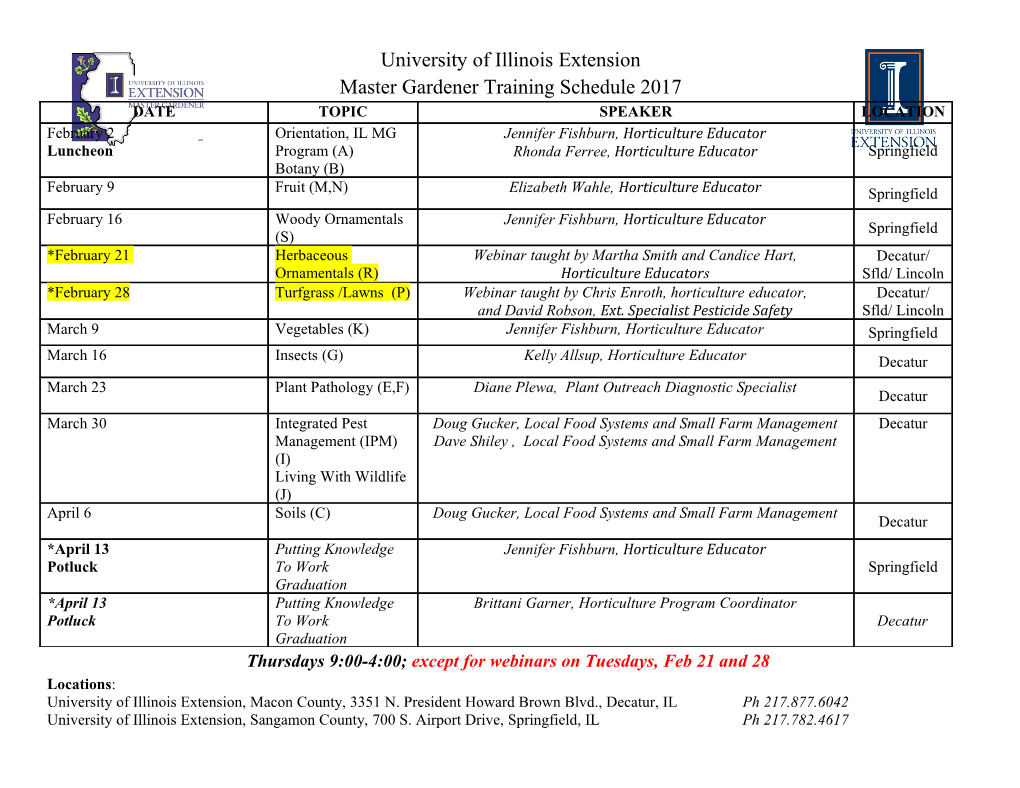
Phys 7221 Homework #2 Gabriela Gonz´alez September 18, 2006 1. Derivation 1-9: Gauge transformations for electromagnetic potential If two Lagrangians differ by a total derivative of a function of coordinates and time, they lead to the same equation of motion: dF (qi, t) ∂F X ∂F L0(q , q˙ , t) = L(q , q˙ , t) + = L(q , q˙ , t) + + q˙ i i i i dt i i ∂t ∂q j j j as proven in the following lines: ∂L0 ∂L0 ∂F = + ∂q˙i ∂q˙i ∂qi ∂L0 ∂L ∂ dF = + ∂qi ∂qi ∂qi dt d ∂L0 ∂L0 d ∂L ∂L d ∂F ∂ dF − = − + − dt ∂q˙i ∂qi dt ∂q˙i ∂qi dt ∂qi ∂qi dt d ∂F ∂ X ∂ ∂F = + q˙ dt ∂q ∂t j ∂q ∂q i j j i ∂ ∂F X ∂F = + q˙ ∂q ∂t j ∂q i j j ∂ dF = ∂qi dt and thus the equations of motion are the same, derived from either Lagrangian: d ∂L0 ∂L0 d ∂L ∂L − = − dt ∂q˙i ∂qi dt ∂q˙i ∂qi 1 (What goes wrong if F = F (q, q,˙ t)?) A particle moving in an electromagnetic field, with scalar potential φ and vector potential A, has a generalized potential function U = φ − A · v and a Lagrangian 1 L = T − U = mv2 − q (φ − A · v) 2 Consider a gauge transformation for the scalar and vector potentials of the form ∂ψ(r, t) φ0 = φ − ∂t A0 = A + ∇ψ(r, t) The change in potential energy under this transformation is U 0 = φ0 − A0 · v ∂ψ = U − q + ∇ψ · v ∂t ∂ψ dr = U − q + ∇ψ · ∂t dt ∂ψ X ∂ψ dxi = U − q + ∂t ∂xi dt dψ = U − q dt and thus, the new Lagrangian differs from the original one by a total derivative: dψ d(qψ) L0 = T − U 0 = T − U + q = L + dt dt and thus we know the equations of motion are the same. We can also see that the electromagnetic forces (and thus Newton’s equations) are invariant. The forces are derived from the potential, following (1.58): ∂U d ∂U Qi = − + ∂qi dt ∂q˙i If U = q(φ(r, t) − A(r, t) · v), then the force is ∂ d ∂ Qi = −q (φ − A · v) + q (φ − A · v) ∂xi dt ∂vi ∂φ ∂ dA = −q + q (A · v) − q i ∂xi ∂xi dt 2 ∂φ ∂ ∂ X ∂ = −q + q (A · v) − q + x˙ A ∂x ∂x ∂t j ∂x i i j j ∂A F = −q ∇φ + + q∇(A · v) − q(v · ∇)A ∂t ∂A = −q ∇φ + + qv × (∇ × A) ∂t = q(E + v × B) and, as we know, the electric and magnetic fields are invariant under the gauge transformation: ∂A0 E0 = −∇φ0 − ∂t ∂ψ ∂ = −∇(φ − ) − (A + ∇ψ) ∂t ∂t ∂A ∂ψ ∂ = −∇φ − + ∇ − ∇ψ ∂t ∂t ∂t ∂A = −∇φ − ∂t = E B0 = ∇ × A0 = ∇ × (A + ∇ψ) = ∇ × A + ∇ × ∇ψ = ∇ × A = B 2. Problem 1-15: A potential for spin interactions Consider a point particle moving in space under the influence of a force derivable from a generalized potential: U(r, v) = V (r) + σ · L We can derive the generalized forces using (1.58): ∂U d ∂U Qi = − + ∂qi dt ∂q˙i using either Cartesian coordinates (x, y, z) or spherical polar coordinates (r, ψ, θ) for generalized coordinates qi. The first choice will produce the components of the force vector Fi; the generalized forces for any other choice of generalized coordinates (such as spherical coordinates) will be related to the force vector as in (1.49): X ∂rj Q = F · i j ∂q j i 3 This problem is a marathon in vector calculus in different coordinate systems. We first calculate the force vector using Cartesian coordinates: U = V (r) + σ · L = V (r) + σ · (r × mv) X = V (r) + m σl(r × mv)l l X X = V (r) + m σl lmnxmx˙ n l m,n X = V (r) + m lmnσlxmx˙ n l,m,n ∂U X = m lmiσlxm ∂x˙ i l,m d ∂U X = m lmiσlx˙ m = (σ × p)i dt ∂x˙ i l,m ∂U ∂V (r) X = + m linσlx˙ n ∂xi ∂xi ln dV (r) ∂r X = − m ilnσlx˙ n dr ∂xi ln dV (r) x = i − (σ × p) dr r i ∂U d ∂U Fi = − + ∂qi dt ∂q˙i dV (r) F = − eˆ + 2(σ × p) dr r Now we want to calculate the generalized forces in spherical polar coordinates r, ψ, θ. We first need to express the potential in terms of the coordinates and their derivatives. We will use unit vectors eˆr, eˆψ, eˆθ, which are themselves functions of the coordinates and time: eˆr = sin ψ cos θˆi + sin ψ sin θˆj + cos ψkˆ ˆ eˆψ = cos ψ cos θˆi + cos ψ sin θˆj − sin ψk eˆθ = − sin θˆi + cos θˆj They are orthogonal and cyclical: eˆi × eˆj = ijkeˆk but they are not constant: in general ∂eˆi/∂qj 6= 0, and also deˆi/dt 6= 0. 4 We first calculate the angular momentum in spherical coordinates: r = reˆr d v = (reˆ ) =r ˙eˆ + rψ˙eˆ + r sin ψθ˙eˆ dt r r ψ θ L = r × p = r × mv ˙ ˙ = mreˆr × (r ˙eˆr + rψeˆψ + r sin ψθeˆθ) 2 ˙ ˙ = mr (ψeˆθ − sin ψθeˆψ) so the potential, in terms of generalized coordinates r, ψ, θ is 2 ˙ ˙ U = V (r) + σ · L = V (r) + mr σ · (ψeˆθ − sin ψθeˆψ) We can now calculate the generalized forces: ∂U d ∂U ∂U Q = − + = − r ∂r dt ∂r˙ ∂r dV = − − 2mrσ · (ψ˙eˆ − sin ψθ˙eˆ ) dr θ ψ dV 2 dV = − − σ · L = − + 2eˆ · (σ × p) dr r dr r ∂U d ∂U Qψ = − + ∂ψ dt ∂ψ˙ ∂ d = −mr2σ · (ψ˙eˆ − sin ψθ˙eˆ ) + (mr2σ · eˆ ) ∂ψ θ ψ dt θ 2 ˙ ˙ 2 ˙ = −mr σ · (− cos ψθeˆψ + sin ψθeˆr) + 2mrrσ˙ · eˆθ − mr σ · θ(sin ψeˆr + cos ψeˆψ) 2 ˙ = −2mr sin ψθσ · eˆr + 2mrrσ˙ · eˆθ ˙ = 2mrσ · (r ˙eˆθ − r sin ψθeˆr) = −2mrσ · (eˆψ × v) = 2reˆψ · (σ × p) ∂U d ∂U Qθ = − + ∂θ dt ∂θ˙ ∂ d = −mr2σ · (ψ˙eˆ − θ˙ sin ψeˆ ) + (−mr2 sin ψσ · eˆ ) ∂θ θ ψ dt ψ 2 ˙ ˙ = −mr σ · (ψ(− sin ψeˆr − cos ψeˆψ) − θ sin ψ(cos ψeˆθ)) 5 2 ˙ 2 ˙ ˙ −m(2rr˙ sin ψσ · eˆψ + r ψ cos ψσ · eˆψ + r sin ψσ · (−ψeˆr + θ cos ψeˆθ)) 2 ˙ = 2mr ψ sin ψσ · eˆr − 2mrr˙ sin ψσ · eˆψ ˙ = 2mr sin ψσ · (rψeˆr − r˙eˆψ) = −2mr sin ψσ · (eˆθ × v) = 2r sin ψeˆθ · (σ × p) Now, we will prove that the force vector F = Fxˆi + Fyˆj + Fzkˆ and the generalized forces Qr,Qψ,Qθ as obtained above, are related by the expression (1.49), using the expression we derived earlier for the force vector: ∂er Qi = F · ∂qi dV (r) ∂ = − ˆr + 2(σ × p) · (reˆr) dr ∂qi dV (r) ∂ Q = − eˆ + 2(σ × p) · (reˆ ) r dr r ∂r r dV (r) = − ˆr + 2(σ × p) · eˆ dr r dV (r) = − + 2eˆ · (σ × p) dr r dV (r) ∂ Q = − eˆ + 2(σ × p) · (reˆ ) ψ dr r ∂ψ r dV (r) = − ˆr + 2(σ × p) · (reˆ ) dr ψ = 2reˆψ · (σ × p) dV (r) ∂ Q = − eˆ + 2(σ × p) · (reˆ ) θ dr r ∂θ r dV (r) = − ˆr + 2(σ × p) · (r sin ψeˆ ) dr θ = 2r sin ψeˆθ · (σ × p) Part(c) of this problem was not included in the homework set, but it maybe the most interesting one: the equations of motion of the system are, using spherical polar coordinates: 6 1 L = T − U = m(r ˙2 + r2ψ˙2 + r2 sin2 ψθ˙2) − V (r) − mr2σ · (ψ˙eˆ − sin ψθ˙eˆ ) 2 θ ψ d ∂L ∂L 0 = − dt ∂q˙j ∂qj d ∂T ∂T d ∂U ∂U = − − + dt ∂q˙j ∂qj dt ∂q˙j ∂qj d ∂T ∂T Qj = − dt ∂q˙j ∂qj We can use the expressions we obtained earlier for the generalized forces. We need to express the equations in terms of the cartesian components of the vector σ, since those are constants (the spherical components are not constants, since the spherical unit vectors themselves are not constant). Since the vector σ is constant, we can choose the z-axis along the σ vector without any loss of generality. Then eˆj · (σ × p) = mσ · (v × eˆj) ˙ ˙ = mσ · (r ˙eˆr + rψeˆψ + r sin ψθeˆθ) × eˆj ˙ ˙ eˆr · (σ × p) = mσ · (−rψeˆθ + r sin ψθeˆψ) = −mσrθ˙ sin2 ψ ˙ eˆψ · (σ × p) = mσ · (r ˙eˆθ − r sin ψθeˆr) = −mσrθ˙ sin ψ cos ψ ˙ eˆθ · (σ × p) = mσ · (−r˙eˆψ + rψeˆr) = mσ(−r˙ sin ψ + rψ˙ cos ψ) d ∂T ∂T Q = − r dt ∂r˙ ∂r dV (r) − + 2eˆ · (σ × p) = mr¨ − mr(ψ˙2 + sin2 ψθ˙2) dr r dV (r) − − 2mσrθ˙ sin2 ψ = mr¨ − mr(ψ˙2 + sin2 ψθ˙2) dr dV (r) mrψ˙2 + mr sin2 ψθ˙(θ˙ − 2σ) = mr¨ + dr d ∂T ∂T Qψ = − dt ∂ψ˙ ∂ψ 7 ˙ 2 ¨ 2 ˙2 2reˆψ · (σ × p) = (2mrr˙ψ + mr ψ) − mr sin ψ cos ψθ −2mσr2θ˙ sin ψ cos ψ = 2mrr˙ψ˙ + mr2ψ¨ − mr2 sin ψ cos ψθ˙2 rθ˙ sin ψ cos ψ(θ˙ − 2σ) = 2rψ ˙ + rψ¨ d ∂T ∂T Qθ = − dt ∂θ˙ ∂θ 2 ˙ 2 ˙ 2 2 ¨ 2reˆθ · (σ × p) = 2mrr˙ sin ψθ + 2mr sin ψ cos ψθ + mr sin ψθ mσ(−r˙ sin ψ + rψ˙ cos ψ) = mr sin ψ(2r ˙θ˙ sin ψ + 2rθ˙ cos ψ + rθ¨sin ψ) 3. Problem 1-19: Spherical pendulum A spherical pendulum is a mass point m suspended by a rigid weightless rod of length l. We set up a coordinate system with the origin at the attachment point, and the z-axis pointing down, along the gravitational force. In spherical polar coordinates, the position vector of the mass is r = leˆr, its velocity is ˙ ˙ v = lψeˆψ + l sin ψθeˆθ, its kinetic energy is 1 1 T = mv2 = ml2(ψ˙2 + sin2 ψθ˙2), 2 2 and the potential energy is V = −mg · r = −mgl cos ψ.
Details
-
File Typepdf
-
Upload Time-
-
Content LanguagesEnglish
-
Upload UserAnonymous/Not logged-in
-
File Pages13 Page
-
File Size-