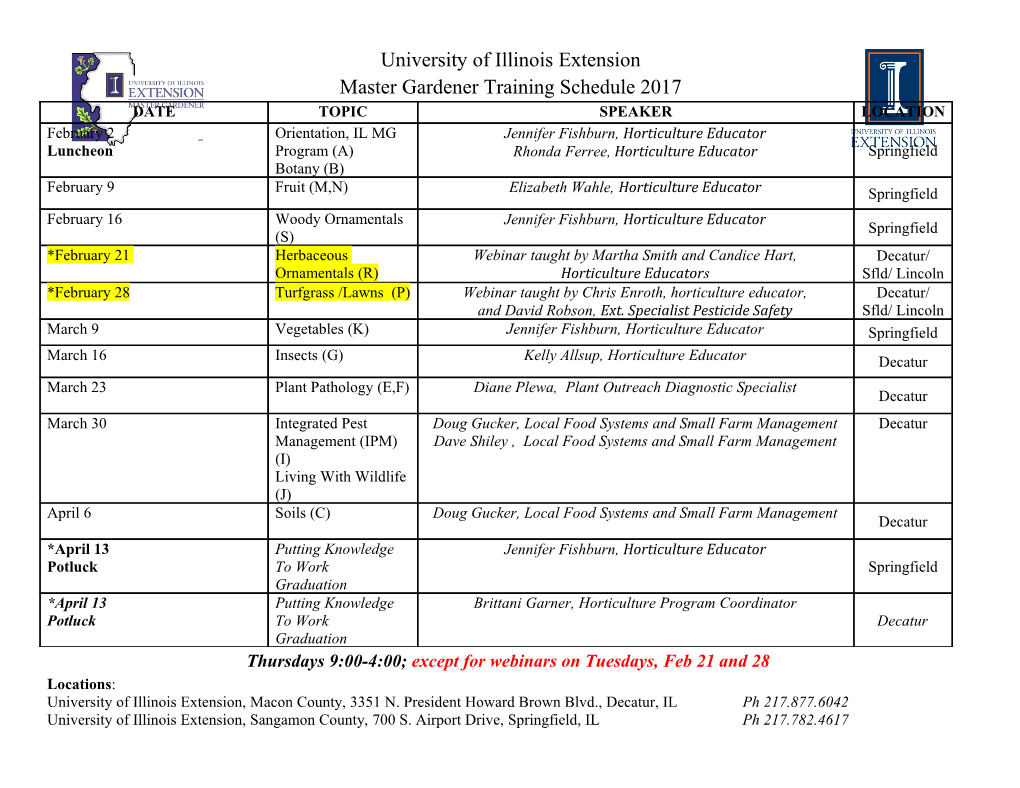
Article Cite This: J. Phys. Chem. A 2017, 121, 8086-8096 pubs.acs.org/JPCA Characterizing Chemical Similarity with Vibrational Spectroscopy: New Insights into the Substituent Effects in Monosubstituted Benzenes Yunwen Tao,† Wenli Zou,‡ Dieter Cremer,†,¶ and Elfi Kraka*,† † Department of Chemistry, Southern Methodist University, 3215 Daniel Avenue, Dallas, Texas 75275-0314, United States ‡ Institute of Modern Physics, Northwest University, Xi’an, Shaanxi 710069, People’s Republic of China ABSTRACT: A novel approach is presented to assess chemical similarity based the local vibrational mode analysis developed by Konkoli and Cremer. The local mode frequency shifts are introduced as similarity descriptors that are sensitive to any electronic structure change. In this work, 59 different monosubstituted benzenes are compared. For a subset of 43 compounds, for which experimental data was available, the ortho-/para- and meta-directing effect in electrophilic aromatic substitution reactions could be correctly reproduced, proving the robustness of the new similarity index. For the remaining 16 compounds, the directing effect was predicted. The new approach is broadly applicable to all compounds for which either experimental or calculated vibrational frequency information is available. ■ INTRODUCTION to the fact that the wave function and electron density12 contain Chemical similarity is an important concept widely used in the all of the information related to energy about a molecule in fields of medicinal chemistry1 and toxicology.2 The origin of question. Quantum chemical descriptors can be divided into this term or its synonym as molecular similarity was greatly two major categories, which describe either the overall influenced by the similarity property principle (SPP), which molecule including HOMO and LUMO energies, dipole states that “similar compounds can have similar properties”,3 moments, total energy, heat of formation, and ionization 4−6 potential or the fragments/substituents of a molecule like the although this does not hold in many scenarios. Maggiora 11 and his co-workers suggested differentiating between chemical net atomic charge. Apart from these, Carbo and co-workers 1 developed a similarity index based on the superposition of the similarity and molecular similarity, where the former stresses ff 13 the physicochemical characteristics of a chemical compound densities of two di erent molecules. Hodgkin proposed a while the latter emphasizes the structural and topological similar approach to measure similarity based on the electro- − 14 properties.7 9 In this work, we will adopt this nomenclature static potential. However, all of the above methods failed to and focus on the chemical similarity, which still has a number of give a detailed and mechanistic description of similarity based open questions to be answered. on the electronic structure, and they are not connected to Chemists tend to put atoms or molecules with similar chemical intuition from which a better understanding can be physicochemical characteristics in the same category in order to obtained. After Bader and his co-workers developed the atoms in generalize empirical rules for practical use. A famous example is 15,16 the periodic table of elements. The physicochemical properties molecules (AIM) theory, Popelier proposed a new method used to characterize chemical similarity are often macroscopic called quantum topological molecular similarity (QTMS), which has been quite successful in characterizing similarity quantities that are measurable, including the pKa, solubility, 17,18 boiling point, octanol−water partition coefficient (log P) and based on chemical insight. Within the framework of QTMS, Popelier constructed the bond critical point (BCP) so forth. Many of these quantities are very important in − quantitative structure−activity relations (QSARs). Since the space, in which any BCP denoted as rb can have 3 8 1960s, the rapid development of quantum chemical methods descriptors derived from the density information at that point, ρ ∇2ρ including the electron density b, the Laplacian of density b, based on quantum mechanics (QM) has made it possible to ϵ calculate the electronic structure and associated properties of the ellipticity of the density b, three connected Hessian molecules, even for large systems with chemical accuracy.10 Many attempts2,11 have been made to develop descriptors or Received: August 19, 2017 models for the characterization of the molecular similarity Revised: September 28, 2017 based on the results of quantum chemical calculations, referring Published: September 29, 2017 © 2017 American Chemical Society 8086 DOI: 10.1021/acs.jpca.7b08298 J. Phys. Chem. A 2017, 121, 8086−8096 The Journal of Physical Chemistry A Article σ Figure 1. (a) Schematic representation of a monosubstituted benzene molecule. (b) xz plane in a C2v monosubstituted benzene. (c) Movement of atoms in the C−H bond stretching mode, C−C−C angle bending mode, and pyramidalization mode. λ λ λ eigenvalues of the density 1b, 2b, and 3b, and the kinetic analysis was done with the program package CO- energy densities K and G . The application of this approach LOGNE2017.37 The diagrams of hierachical clustering analysis b b − has been summarized in several articles.19 22 However, QTMS were generated with the software package SPSS 23.38 has some limitations that might hinder its general usefulness. (i) The number of descriptors depends on the number of ■ RESULTS AND DISCUSSION chemical bonds or noncovalent interactions that have BCPs. In the following part, the outcome of our study will be Considering the chemical bonds exclusively may fail in discussed. capturing all information on the electronic structure. (ii) The Similarity of the Monosubstituted Benzenes. Before descriptors are heterogeneous with regard to the physical describing the similarity of the benzene derivatives, it is meaning and have different units. A horizontal comparison necessary to give a brief introduction into the theory of the 23,39 between the descriptors does not make sense. (iii) QTMS local vibrational modes. For any internal coordinate qn fi requires specialized procedures in the feature selection step, speci ed within a molecule, its local mode vector an is given by which may involve the principal component analysis (PCA), in fi Kd−†1 order to nd the most important descriptors. a = n In this work, we present a new approach to measure chemical n −†1 dKnn d (1) similarity based on the theory of local vibrational modes, which was originally proposed by Konkoli and Cremer.23 The local where K is the force constant matrix transformed into normal † x vibrational modes can be explained with the leading parameter coordinates Q, K = L f L. dn is a row vector of the D matrix, principle. The motions of a local mode vector are obtained after which collects the normal modes in terms of internal relaxing all parts of the vibrating molecule except an internal coordinates, D = BL. The matrix L contains all normal mode coordinate parameter (leading parameter), which is first vectors in Cartesian coordinates obtained by solving the Wilson displaced infinitesimally, e.g., bond stretching, angle bending, equation of vibrational spectroscopy, while the Wilson B matrix dihedral torsion, out-of-plane torsion, and so forth. With a well- is used to connect Cartesian coordinates to internal 40 established physical basis, this approach is free from the coordinates. a disadvantages of the QTMS method and is able to access the The local mode force constant kn can be obtained by information on the electronic structure by examining different † k a = aKa types of internal parameters besides chemical bonds. Therefore, n n n (2) this can be regarded as an extension of our previous With the help of the G-matrix,23 the reduced mass of local 24−33 fi studies focused on chemical bonding. We apply in this vibrational mode an can be de ned. Thus, the local vibrational ωa work our new similarity measure to a test set of 60 frequency n is determined by monosubstituted benzene molecules. The paper is structured a 2 1 a in the following way: After summarizing the computational (ωn ) = 22kGn nn methods used, in the results and discussion part, the basic 4π c (3) theory of the local vibrational modes and the use of local mode Normal and local vibrational modes are second-order frequencies as similarity descriptors of benzene derivatives are response properties;41 therefore, they are very sensitive to described. Then, we discuss the directing effects of the any change of the electronic structure. That is the reason why substituents on benzene in electrophilic aromatic substitution chemists have been intensively using vibrational spectroscopy reactions. Conclusions are given in the last part. for structural characterization. In order to study the electronic structure of different ■ COMPUTATIONAL METHODS monosubstituted benzene molecules, we treat the substituent as Geometry optimization and normal mode analysis for all a perturbation of the phenyl ring to which it is linked and use benzene derivatives involved in this work were carried out using benzene as the reference. the ωB97X-D density functional34 with Dunning’s aug-cc- By doing so, any perturbation of the targeted system can be pVTZ basis set35 in the Gaussian09 package.36 The local mode characterized by the red or blue shift of absorption peaks in 8087 DOI: 10.1021/acs.jpca.7b08298 J. Phys. Chem. A 2017, 121, 8086−8096 The Journal of Physical Chemistry A Article Table 1. Comparison of Calculated Vibrational
Details
-
File Typepdf
-
Upload Time-
-
Content LanguagesEnglish
-
Upload UserAnonymous/Not logged-in
-
File Pages11 Page
-
File Size-