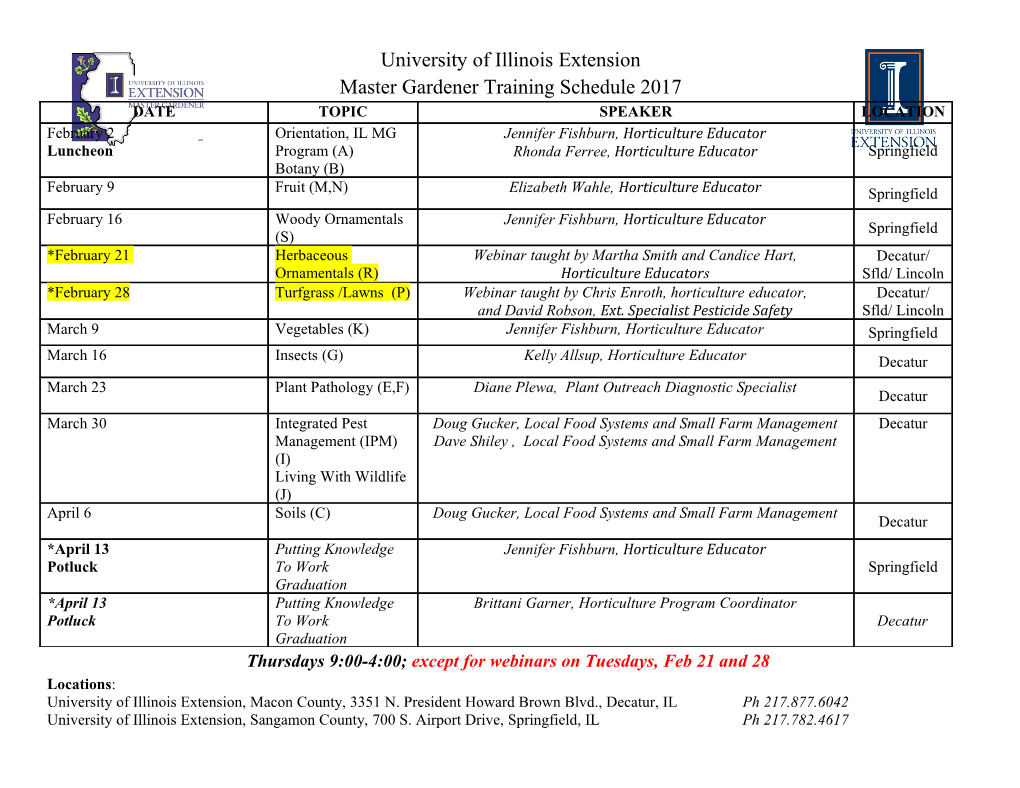
Systems Engineering at the Nanoscale Jennifer L. Sample and Harry K. Charles Jr. anotechnology has become ubiquitous in advanced technology and advanced product development. Nanotechnology exploits the properties of materials with at least one dimension at the nanoscale, typically in a novel and controllable way. As a result, nanotechnology has the ability to affect materials and structures as part of complex systems that can influence actions in the macroscopic world. This article explores nanotechnology and its emergence into systems engineering, highlighting attributes of the technology that may play a significant role in APL-developed systems of the future. INTRODUCTION A system is a composite of different elements that Once the properties of nanostructures are well charac- when brought together yield results that are not obtain- terized and can be reproducibly fabricated, those proper- able from the elements alone.1 The function of systems ties can be exploited as a technology. engineering is to guide the development of individual Two important concepts define a system: components into robust and efficient systems capable 1. The system has properties or attributes that cannot of autonomous operation as complex systems, where be associated with any of its components.2 complex systems are defined as systems in which ele- ments are diverse and have intricate relationships with 2. The description of the system function is less com- each another. Nanotechnology refers to the creation of plex than the functional descriptions of the system components. These concepts or properties are called functional materials, devices, and systems via control 3 of matter on the nanometer-length scale (nominally emergent properties of the system. 1–100 nm) and exploitation of novel phenomena and Emergence in the engineering disciplines is an properties (physical, chemical, biological) at that length expected outcome. Emergence can also occur in nano- scale. In general, nanotechnology begins with nanosci- material systems. ence: the study of material properties and their interac- For example, a microprocessor chip system can per- tions at the nanoscale and how they can be exploited. form millions of arithmetical functions per second 50 JOHNS HOPKINS APL TECHNICAL DIGEST, VOLUME 31, NUMBER 1 (© 2012) under precise control of a software program. These ing (MESSENGER) probe, a mission to the planet Mer- mathematical functions are a straightforward manifes- cury. Naturally occurring complex systems can range tation of the input and output behavior of the software from biological entities to meteorological events. Virtu- code. This description is much simpler than a descrip- ally all important properties of engineered systems are tion of the microprocessor chip states through which emergent. The primary difference between engineered the chip would pass as the function is computed under and naturally occurring systems is that in human-pro- the direction of the software code. Such component duced systems, the emergent properties are produced descriptions—regardless of the level considered, from intentionally. Yet other real differences exist: human- individual quantum levels to gate or machine language engineered systems are often functionally fragile and do functions—are much more complex than the descrip- not hold up well in the environment in which they are tion of the mathematical function itself. Microproces- used, whereas natural systems seem to be more robust, sors, however, are typically part of multiscale systems flexible, and adaptable. These differences are due in such as cellular phones and automotive sensors and part to the top-down or hierarchical design approach3 similarly could be part of systems linking nanotechnol- used in human-engineered systems. At each level, per- ogy to society. formance goals are set and then subcomponents are This article will describe the development of nano- added with the needed properties to achieve the per- technology for small systems or components at APL. formance at the next upward level. For each of the sub- Examples include physiologically responsive polymers, components, the same is done with sub-subcomponents which release drugs only in response to a need-based and so on. physiological stimulus;4 nanoporous DNA sensors, In contrast, nature designs systems from the bottom which electrically detect DNA segments binding to a up. Existing or modified components are put together known segment;5 and biologically generated nanoscale with no known performance constraints or functionality cellulose composites, which provide strong transparent goals in mind. The resultant system (assemblage) either materials for structural or biomedical applications.6 All survives in the environment or dies (trial and error and of these technologies are currently under development survival of the fittest). Nature’s designs are not always at APL. perfect, but surviving systems perform the required functions, and nature does not have to work against budget and schedule constraints. The description in this COMPLEX SYSTEMS article of the latest developments in microelectronics Complex systems or complex adaptive systems have and nanotechnology explores the application of nature- been studied extensively for almost 40 years.7 They emulating systems built by the self-assembly of funda- trace their origins to the beginnings of chaos theory in mental components versus the top-down approach of 1890 by Jules Henri Poincaré8 (1854–1912), a professor conventional human-engineered systems. Both system of mathematics at the University of Paris from 1881 to types play important roles in all areas of science and 1912, and to the work in the 1960s of Edward Norton engineering development. Lorenz9 (1917–2008), mathematician, meteorologist, and professor at Massachusetts Institute of Technol- ogy. To define a complex system we must consider the NANOTECHNOLOGY concept of upward predictability. Upward predictability The properties of nanoscale materials differ from implies that an analysis of interactions at the compo- their macroscopic counterparts. One ubiquitous differ- nent level will yield a simple explanation of the phe- ence is higher surface area; nanostructures can have as nomena observed at the system level. Systems that can much as 90% of the atoms at the surface (Fig. 1). be analyzed in this manner are upwardly predictable. This increased surface area leads to improved cata- Complex systems often are not upwardly predictable. lytic performance at the nanoscale for catalytic materi- The effects of interactions at the component level are als. It also leads to a maximization of relevant surface correlated in complex ways to the phenomena occurring effects, such as capillary forces and electrostatic attrac- at the system level. This lack of upward predictability, tion, which can be exploited to assemble nanoparticles coupled with emergence concepts, is the hallmark of a into functional, hierarchical arrays.10 complex system. Variance in optical properties with nanomaterial size Complex systems can be engineered by humans and shape has been known for centuries in the use of gold or they can occur naturally. Representative human- and silver nanoparticles as pottery glazes and stained- engineered systems include many APL systems, includ- glass pigments.11 Gold and silver exhibit size-dependent ing missile defense and spacecraft systems such as the optical properties because they support a surface plas- Standard and Tomahawk missiles, the Midcourse Space mon—a collective oscillation of free electrons in the Experiment (MSX, a DoD satellite), and the MErcury material that can resonate, absorbing light in the visible Surface, Space ENvironment, GEochemistry and Rang- spectrum. In recent decades, these materials have found JOHNS HOPKINS APL TECHNICAL DIGEST, VOLUME 31, NUMBER 1 (© 2012) 51 J. L. SAMPLE AND H. K. CHARLES JR. exploit the self-assembling material properties of DNA Macro to enable a small “robot” to perform functions such as 1 cm walking down a track.16 APL researchers have developed functional nano- materials and devices to address critical challenges 1 mm in homeland protection. For example, one project, the Nanoporous DNA Sensor, uses nanotechnol- 0.1 mm ogy to achieve rapid, straightforward DNA matching. Nanoscale pores are functionalized with complementary Micro DNA, and the size of the pores is small enough that DNA 10 m binding inside the pore will change impedance through the porous membrane in a detectable way. This technol- ogy is envisioned as an alternative to DNA microarray 1 m technology, providing the advantage of reduced sample Size scale preparation requirements, which will be useful in rapid screening scenarios. Another APL project uses electro- 0.1 m deposited bismuth nanowires to detect radiation. Inci- Nano dent gamma rays generate detectable electrical current in these materials. These bismuth nanowire arrays have 10 nm the significant advantage of being less expensive to pro- duce than the current technology, which involves large single-crystal semiconductor detectors. In addition to 1 nm these sensor nanowire applications, metal nanotubes are being investigated at APL for high-energy-density thin- 0.1 nm film battery applications. These thin-film battery nano- tubes are filled with battery chemicals, and they can be 0 20 40 60 80 100 geometrically engineered to minimize battery inefficien- Atoms at the surface (%) cies such as diffusion-limited transport between anode and cathode.17 Figure 1. Nanoscale
Details
-
File Typepdf
-
Upload Time-
-
Content LanguagesEnglish
-
Upload UserAnonymous/Not logged-in
-
File Pages8 Page
-
File Size-