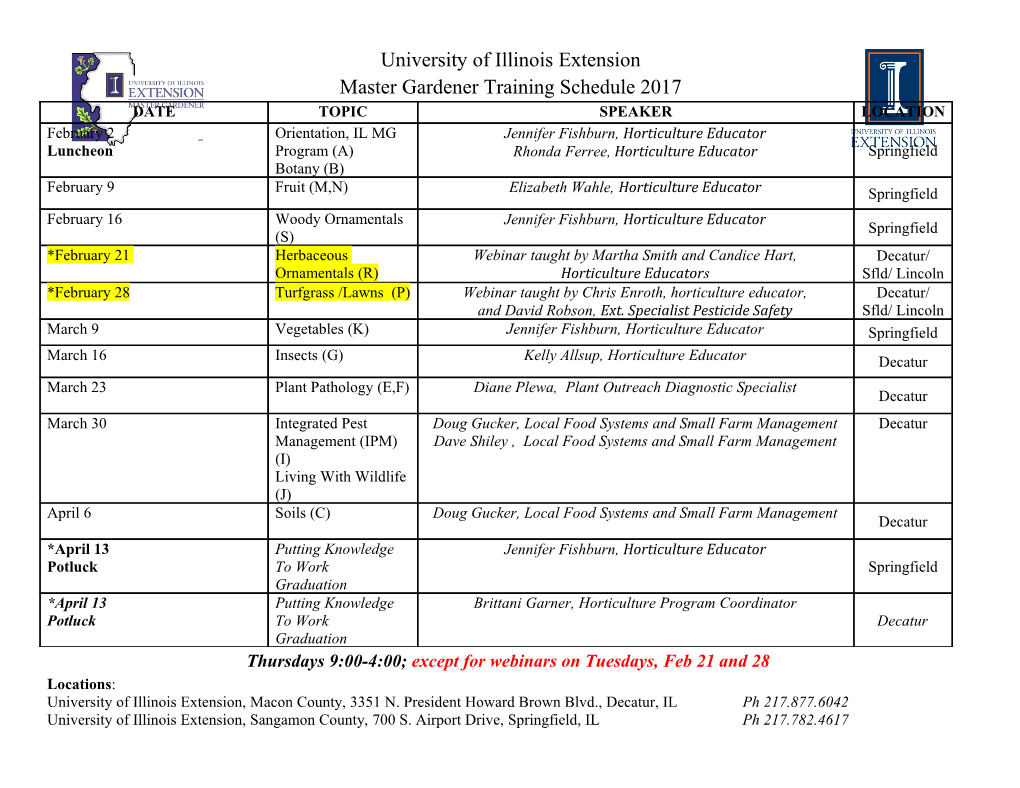
Chemical kinetics modelling of combustion processes in SI engines by Ahmed Faraz Khan B.Sc., M.S. Submitted in accordance with the requirements for the degree of Doctor of Philosophy The University of Leeds School of Mechanical Engineering May 2014 The candidate confirms that the work submitted is his own, except where work which has formed part of jointly authored publications has been included. The contribution of the candidate and the other authors to this work has been ex- plicitly indicated below. The candidate confirms that appropriate credit has been given within the thesis where reference has been made to the work of others. The Figure 2.5 in Chapter 2 has been published in a jointly authored publication: A.F.Khan and A.A.Burluka. An Investigation of Various Chemical Kinetic Models for the Prediction of Autoignition in HCCI Engine. ASME 2012 Internal Combustion Engine Division Fall Technical Conference, Vancouver, BC, Canada, September 23 – 26, 2012. Paper No. ICEF2012-92057, pp. 737-745; doi:10.1115/ICEF2012-92057 The content taken from the above publication for use in this thesis was solely generated by the author. The co-author is credited to technical discussion on the results produced in the publication. This copy has been supplied on the understanding that it is copyright material and that no quotation from the thesis may be published without proper acknowl- edgement. The right of Ahmed Faraz Khan to be identified as Author of this work has been asserted by him in accordance with the Copyright, Designs and Patents Act 1988. c 2014 The University of Leeds and Ahmed Faraz Khan i Acknowledgements The completion of this work owes a lot to the contribution and support of so many people in my professional and personal life. This is where I show my gratitude and acknowledge their support which is certainly immeasurable in words. First and foremost, my supervisor Dr Alexey Burluka, my utmost thanks for the guidance all through the years – you have always been there. In addition to all-things-combustion, conversations on world politics were indeed enlightening. I also enjoyed the crash course on how to appreciate opera while driving down to Northampton – I have seen a couple of them after that with tremendous enjoyment. But above all, many thanks for giving me the opportunity to do this PhD. Unfortunately, I did not have much interaction with Prof Chris Shep- pard due to his retirement but his spirit was certainly present in the form of a plethora of SAE papers and titbits from Alex. My utmost gratitude to a bunch of brilliant engineers and fantastic human beings at Mahle Powertrain. Without any exaggeration, work- ing with you guys was one of the most enjoyable aspects of my PhD. I have to name some names here: Jens Neumeister, Dave Oudenijew- eme and Paul Freeland, many many thanks for helping me on so many things. I certainly learned a lot from you guys. Thanks for keeping me on track too, those weekly teleconferences were very useful, not sure about the Gantt charts though. A special thanks must also go to John Mitcalf for taking the time out for all the CFD work, thanks to David Gurney and Andre Bisordi for tips on GT-Power. I must acknowledge Prof Jeffrey Cash at Imperial College London for the numerical library MEBDFI, Prof Valeri Golovitchev at Chalmers Institute of Technology for kindly providing the gasoline mechanism and Dr Youngchul Ra at University of Wisconsin-Madison for the Re- itz/MultiChem mechanism. Due thanks to Dr Phil Roberts for the permission to use LUPOE2-D experimental data. Colleagues in combustion group at Leeds University, who are (in no particular order) Tawfiq, Navanshu, Ahmed, Dominic, Zhengyang, Nini, Daniel, it was a great pleasure working with you all – my best wishes for your future. I must pay a special tribute to Dr Graham Con- way who always took the time out to attend to my questions, many thanks for all those discussions on LUSIE and other things in general. Your presence made things a lot easier for me in the early days. Thanks to the IT staff at the School, in particular, John Hodrien, whose help always came with words of wisdom on all-things-LINUX. My housemates over the years who became my BFFs, Sabina, Lubka, Eva, Danny, Julian, Sandra and Beta, I could not have asked for better friends – thank you! BTW I wouldn’t have to do a PhD if I had a penny for every time you asked me, “when do you finish?”. Lastly, because it’s the most important one, my family. I have no words to thank you with for the limitless love and support you have given me over the years. Ami, Abu, Khurram, Ambreen, Babar, Aleezah, thank you all! Abstract The need for improving the efficiency and reducing emissions is a constant challenge in combustion engine design. For spark ignition engines, these challenges have been targeted in the past decade or so, through ‘engine downsizing’ which refers to a reduction in engine displacement accompanied by turbocharging. Besides the benefits of this, it is expected to aggravate the already serious issue of engine knock owing to increased cylinder pressure. Engine knock which is a consequence of an abnormal mode of combustion in SI engines, is a performance limiting phenomenon and potentially damaging to the engine parts. It is therefore of great interest to develop capability to predict autoignition which leads to engine knock. Traditionally, rather rudimentary skeletal chemical kinetics models have been used for au- toignition modelling, however, they either produce incorrect predic- tions or are only limited to certain fuels. In this work, realistic chemi- cal kinetics of gasoline surrogate oxidation has been employed to ad- dress these issues. A holistic modelling approach has been employed to predict com- bustion, cyclic variability, end gas autoignition and knock propen- sity of a turbocharged SI engine. This was achieved by first devel- oping a Fortran code for chemical kinetics calculations which was then coupled with a quasi-dimensional thermodynamic combustion modelling code called LUSIE and the commercial package, GT-Power. The resulting code allowed fast and appreciably accurate predictions of the effects of operating condition on autoignition. Modelling was validated through comparisons with engine experimental data at all stages. Constant volume chemical kinetics modelling of the autoignition of various gasoline surrogate components, i.e. iso-octane, n-heptane, toluene and ethanol, by using three reduced mechanisms revealed how the conversion rate of relatively less reactive blend components, toluene and ethanol, is accelerated as they scavenge active radical formed during the oxidation of n-heptane and iso-octane. Autoigni- tion modelling in engines offered an insight into the fuel-engine inter- actions and that how the composition of a gasoline surrogate should be selected. The simulations also demonstrated the reduced relevance of research and motor octane numbers to the determination of gaso- line surrogates and that it is crucial for a gasoline surrogate to reflect the composition of the target gasoline and that optimising its physic- ochemical properties and octane numbers to match those of the gaso- line does not guarantee that the surrogate will mimic the autoignition behaviour of gasoline. During combustion modelling, possible deficiencies in in-cylinder tur- bulence predictions and possible inaccuracies in turbulent entrain- ment velocity model required an optimisation of the turbulent length scale in the eddy burn-up model to achieve the correct combustion rate. After the prediction of a correct mean cycle at a certain engine speed, effects of variation in intake air temperature and spark timing were studied without the need for any model adjustment. Autoigni- tion predictions at various conditions of a downsized, turbocharged engine agreed remarkably well with experimental values. When cou- pled with a simple cyclic variability model, the autoignition predic- tions for the full spectrum of cylinder pressures allowed determina- tion of a percentage of the severely autoigniting cycles at any given spark timing or intake temperature. Based on that, a knock-limited spark advance was predicted within an accuracy of 2◦ of crank angle. Contents Contentsv List of Figures ix List of Tables xvii Nomenclature xix 1 Introduction to topic and terminologies1 1.1 Introduction and motivation . .1 1.2 Scope of this work . .3 1.3 Thesis organisation . .4 1.4 Autoignition and knock . .5 1.4.1 Factors affecting engine knock . .6 1.4.1.1 Engine design and operating conditions . .6 1.4.1.2 Fuel effects . .8 1.5 Ignition delay time measurements . 10 1.6 Autoignition modelling . 12 2 Principles and modelling of chemical kinetics 15 2.1 Introduction . 15 2.2 Chemical kinetics fundamentals . 16 2.2.1 Rate of formation or depletion of species . 18 2.2.1.1 Three-body collision reactions . 19 2.2.2 Pressure dependant or fall-off reactions . 20 2.2.3 Thermodynamic properties . 22 2.3 Numerical integration . 23 2.4 Code development . 23 2.5 Chemical kinetics mechanisms . 25 v CONTENTS 2.5.1 Andrae model . 28 2.5.2 Golovitchev model . 29 2.5.3 Reitz model . 30 2.6 Code validation . 30 2.7 Autoignition simulation results . 33 2.8 Practical gasoline surrogates . 36 2.9 Gasoline surrogate formulation . 42 2.10 Summary . 44 3 Hydrocarbon oxidation chemistry 46 3.1 Introduction . 46 3.2 General features of hydrocarbon oxidation . 49 3.2.1 Low temperature oxidation . 49 3.2.2 High temperature oxidation . 53 3.3 Numerical predictions of oxidation pathways . 54 3.3.1 Iso-octane . 54 3.3.2 N-heptane . 57 3.3.3 Toluene . 60 3.3.3.1 Effect of doping on toluene oxidation .
Details
-
File Typepdf
-
Upload Time-
-
Content LanguagesEnglish
-
Upload UserAnonymous/Not logged-in
-
File Pages223 Page
-
File Size-