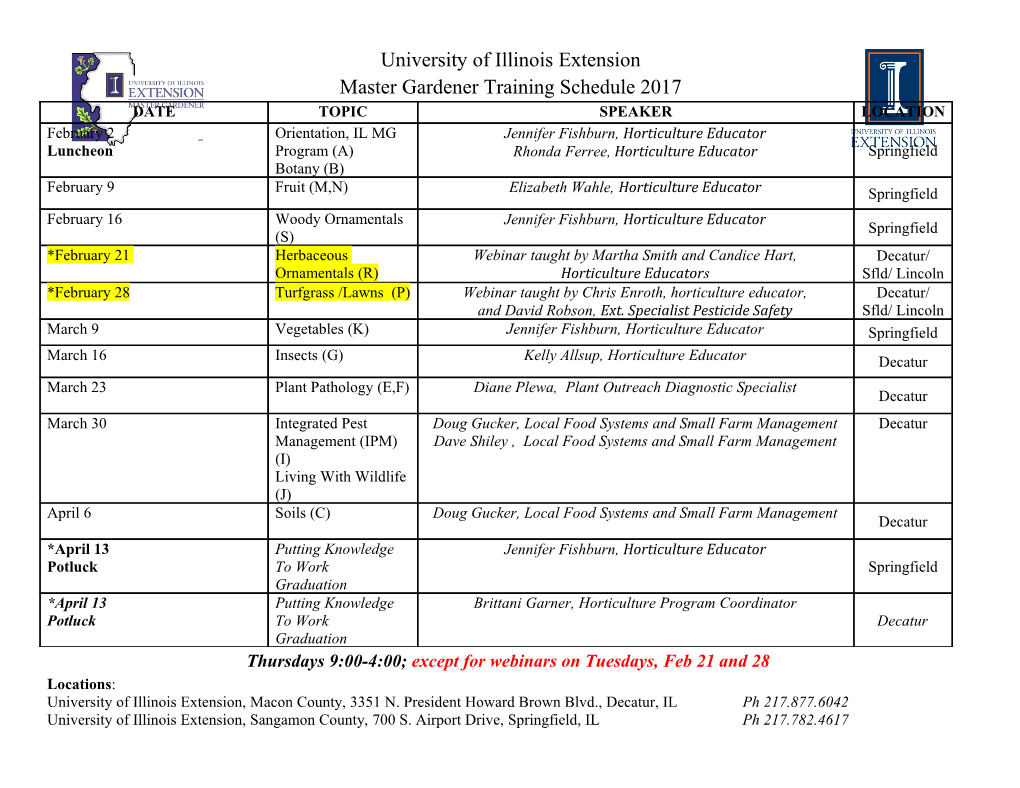
I C Lau Remote Sensing Chapter 4 Remote Sensing Background Introduction Remote sensing is classically defined as the science of acquiring and analysing information about an object or area from a distance. This may be accomplished by the measurement of (1) emitted or reflected electromagnetic (EM) radiation, (2) magnetic or gravity fields, (3) emitted gamma-rays (?-rays) from the decay of radioelements and (4) mechanical vibrations or waves emanating from, being transmitted through or reflected from materials (Reeves 1975). A more modern definition describes remote sensing as the measurement of EM energy by aircraft- or spaceborne-mounted sensors for the interpretation of surface and subsurface features. The purpose of this chapter is to introduce the theory behind remote sensing with emphasis on mineralogical and geological information extraction. The first section of the chapter defines and explains the properties of EM radiation and the propagation of spectral features in the visible-near infrared (VNIR) to shortwave infrared (SWIR) regions and the interaction of radiation with the materials on Earth. The second part of the chapter summaries the acquisition and recording of EM radiation by remote and proximal sensors. The final part of the chapter explains the processing and the extraction of information of remotely sensed data for geoscientific purposes in. Part 1 Electromagnetic Radiation Theory and the Interaction with Materials Background on Electromagnetic Radiation Electromagnetic energy has been defined as both a wave and a particle model and hence, can be defined by frequency or wavelength. The particle model describes EM radiation as discrete packets of energy called photons, Q with joules the unit of measurement. The following equation, Planck’s Law, is used to define EM energy as a photon: Q=hf (1) Where h is Planck’s constant, 6.625x10-34 J sec (=4.14x10-15 eV sec), and f the frequency of the radiation. This can also be expressed as Q=ch/? (2) - 49 - Chapter 4 where c is the speed of light. Thus the higher the energy of the photon, the shorter the wavelength and greater the frequency. The speed of light is given by: c2=1/eµ (3) where the dielectric permittivity e and the magnetic permeability , reduce to eo -12 -1 (dielectric permittivity of a vacuum = 8.85 x 10 Fm ) and µo (magnetic permeability of a vacuum = 12.5 x 10-7 Hm-1) in a vacuum: 2 8 -1 2 c = 1/ eoµo = (3.0x10 ms ) (4) Maxwell’s wave theory describes EM radiation as sine wave motions of oscillating electric and magnetic fields (Figure 4.1). The following general equation can be used to describe the interactions of the wavelength (?) and frequency (f) of the radiation: c=f? (5) The above terms can be used to define the distance between the wave crests (wavelength) and the number of oscillations or vibrations passing one point in a second (frequency) (Figure 4.1). The units used in spectroscopy to measure the wavelength of the EM radiation are commonly given as metres (m), centimetres (cm), micrometres (µm =10-6 m) or nanometres (nm =10-9 m), while frequency is given as hertz (Hz). Figure 4.1 Propagation of EM energy as a wave. Wavelength is defined by the distance between the crests of the waves, the amplitude is the height of the waves and frequency is the number of oscillations through a point on the wave for a period of time (adapted from Avery & Berlin (1992)). Note that electric and magnetic fields are always orthogonal. - 50 - I C Lau Remote Sensing The EM spectrum (Figure 4.2) constitutes a range of different types of EM radiation that correspond to distinct levels of energy. Short wavelength radiation is represented by gamma-rays, which have high frequencies and high quantum energies. Long wavelength EM radiation, such are radio waves, have low frequencies and possess low quantum energies (Figure 4.2). The visible region (VIS, 0.4 - 0.7 µm), which corresponds to the energies observed by the human eye, consists of only a small region of the EM spectrum (see Table 4.1). Figure 4.2 Electromagnetic spectrum of radiation. The visible region has been expanded to display the corresponding wavelengths of reflected colours seen by the human eye (Table 4.1) (Adapted from Nave 2003). As well as the visible region, various remote sensing instruments measure EM radiation in the near-infrared (NIR) to shortwave infrared (0.8 - 2.5 µm), the thermal infrared (TIR) (8 - 13 µm), radar (cm scale wavelength) and g-radiation regions. The utilisation of a wider range of EM spectrum allows a multitude of different physical and chemical properties of materials to be measured. The understanding and discrimination of the spectral features caused by the different physical and chemical properties of matter can be used to assist the identification of the constituents of minerals or molecular substances that constitute the material. - 51 - Chapter 4 Wavelength range (mm) Colour of light absorbed Colour seen by eye 0.4 - 0.42 Violet Green-Yellow 0.42 - 0.44 Violet-Blue Yellow 0.44 - 0.47 Blue Orange 0.47 - 0.5 Blue-Green Red 0.5 - 0.52 Green Purple 0.52 - 0.5 Yellow-Green Violet 0.05 - 0.58 Yellow Violet-Blue 0.58 - 0.62 Orange Blue 0.62 - 0.68 Red Blue-Green Table 4.1 Characteristics of light observed by the human eye. Commonly, remote sensing instruments acquire EM radiation at 0.45 mm, 0.5 mm and 0.65 mm corresponding to blue-green-red colours and are integrated into combinations to produce other colours, such as cyan, magenta, yellow and white. Electronic and Molecular Processes: The Principles of Spectral Absorption Incident EM energy interacts with the materials at the surface of an object. The EM radiation that is reflected possesses information on the physical and chemical composition of its constituents. Comparison of the spectral and EM radiation properties of the measured incident and reflected radiations allow the calculation of the reflectance quantity of the target material over a range of wavelengths. This process is essentially the creation of a plot of values for the amount of reflectance over a range of wavelengths. From this plot, or ‘spectrum’, inferences can be made on the composition of the target material. Grain size and roughness of the surface are the major physical properties that affect the spectral response. The chemical properties of the materials influence the electronic and vibrational processes that occur on a molecular to atomic scale when EM radiation interacts with an object. The electrical and vibrational processes from different atoms and molecular groups that constitute a material affect the reflectance spectrum in a variety of ways. It is the understanding of these two fundamental processes that allow the identification of each absorption feature observed in a measured spectrum. Electronic processes, such as the shift of electrons between orbital shells, generate spectral features in the ultraviolet (UV) and VNIR regions (0.3 - 1.3 µm) (Figure 4.4). Vibrational processes from the subtle movement of molecules or bonded atoms generate spectral features in the infrared (IR) regions (1.4 - 40.0+ µm) (Hunt & Salisbury 1970). Understanding of the cause of the electronic and vibrational processes - 52 - I C Lau Remote Sensing is important for identifying the spectral features produced. Once the causes of the spectral features are identified and the atoms/molecules creating the electronic and vibrational processes are known, then the identification of the possible constituent minerals can be made. Figure 4.3 Interaction of electromagnetic energy with the surficial layers of a material occurs with the top 50 mm. (ii) Reflection occurs from grain surfaces. (iii) A transparent mineral may transmit EMR where it is reflected by the material below, (i) or the energy may reflect off of the bottom of the crystal structure. Each of these processes will influence the nature of the electromagnetic energy recorded by the sensor. Electronic processes –Visible and Near Infrared (VNIR) Spectral Features (0.4 - 1.3 mm) Spectral features in the VIS (0.4 - 0.7 mm) and NIR (0.8 - 1.3 mm) regions are related to electronic processes, involving the energy levels of electrons within the elements that constitute the materials. There are two dominant electronic processes that are of interest in the study of minerals; ligand crystal field effects, and ligand charge transfer effects. A ligand is a dipolar group that binds to a larger molecule or central metal atom and causes a response on the receptor. In the case of spectral studies, the binding of a ligand to a metal atom can be seen as absorption features in the VNIR. - 53 - Chapter 4 The ligand crystal field effect involves the absorption of radiation by unpaired, inner shell electrons of transition metal ions, causing their excitation to outer shells (Burns 1970), as shown in Figure 4.4. The effect is due to the electrostatic fields created by the surrounding anions or dipolar molecules (ligands) that constitute the crystal structure (commonly a lattice silicate) of a mineral. The element Fe, which occurs as ferric (Fe3+) and ferrous (Fe2+) ions, plays an important role in crystal field effects due to its substantial abundance in regolith minerals and its ability to substitute into tetrahedral and octahedral sites. The absorption of short wavelength EM radiation by Fe2+ and subsequent transition of an excited electron into the excited state is accompanied by a diagnostic absorption in the VNIR region, called the crystal field absorption (CFA). Most CFA features seen in spectra are due to Fe3+ and Fe2+ ions. The broad absorption feature in the 0.8 - 1.0 mm region is related to the crystal field electronic process (Figure 4.6).
Details
-
File Typepdf
-
Upload Time-
-
Content LanguagesEnglish
-
Upload UserAnonymous/Not logged-in
-
File Pages61 Page
-
File Size-