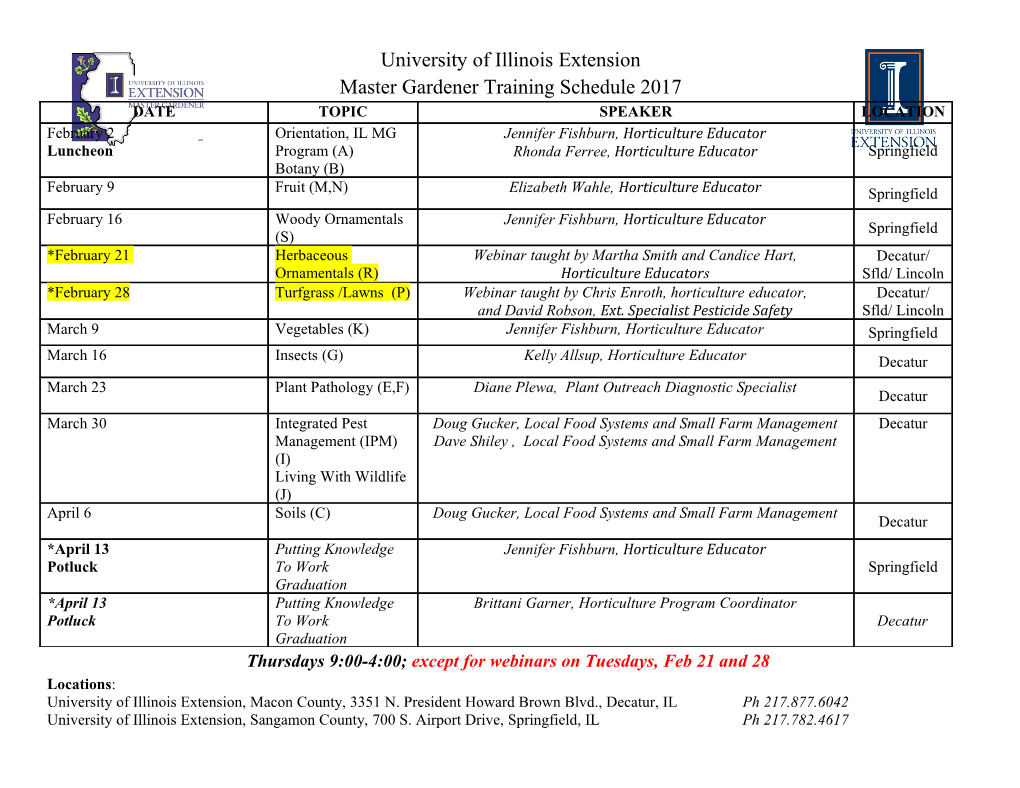
Progress in Drug Research, Vol. 62 (Markus Rudin, Ed.) ©2005 Birkhäuser Verlag, Basel (Switzerland) Magnetic resonance and fluorescence based molecular imaging By David Sosnovik and technologies Ralph Weissleder Center for Molecular Imaging Research, Massachusetts General Hospital, Harvard Medical School, Fruit Street, Boston, MA 02114, USA <[email protected]> Magnetic resonance and fluorescence based molecular imaging technologies Glossary of abbreviations FMT, fluorescence molecular tomography; FRI, fluorescence reflectance imaging MRS, magnetic relaxation switches, NIRF, near infrared fluorescence, MNP, superparamagnetic nanoparticles; MRI, magnetic resonance imaging; PET, positron emission tomography; SNR, sig- nal-to-noise ratio; SPECT, single-photon emission computed tomography. 1 Introduction The traditional role of non-invasive imaging has been to define gross anatomy and physiology at the level of the whole organ. In the last few years, however, technological advances have made it possible to characterize mol- ecular expression and physiology at the cellular level with non-invasive imaging techniques. This emerging field has been broadly termed “molecu- lar imaging” and has the potential to significantly enhance the role of diag- nostic imaging in both the basic science and clinical arenas. This review sum- marizes some of the recent developments in molecular imaging and discusses their potential impact on several important aspects of drug discovery and development. We focus our attention in this chapter on optical (fluores- cence), and magnetic resonance imaging (MRI) approaches to molecular imaging. The role of bioluminescence, single-photon emission computed tomography (SPECT) and positron emission tomography (PET) imaging have been covered elsewhere in this book. For a molecular imaging tool to be useful, however, several prerequisites must be met. It must have a sensitivity high enough to monitor interactions at the molecular level, a sufficiently high spatial resolution to image mouse models of human disease and a high degree of specificity for the target of interest [1]. Each imaging modality has a set of advantages and disadvantages, and the choice of technique must thus be tailored to the question of inter- est. For instance, if high-resolution images of myocardial function and con- traction in a live mouse are desired, MRI is likely to be the modality of choice [2]. On the other hand, activatable near infrared fluorescence (NIRF) probes are highly suitable for the detection of enzyme activity within endothelial membranes and accessible tumors [3, 4]. The use of mouse models of human disease has become increasingly more important in the era of molecular and genetic medicine [5]. Mouse models are frequently used in drug development to validate potential tar- 85 David Sosnovik and Ralph Weissleder gets, to assess therapeutic efficacy, and to identify and validate biomark- ers of drug efficacy and/or safety. The emergence of imaging technology capable of obtaining high quality non-invasive images of mice, in vivo, has thus been critical to the development of molecular imaging [6]. Sys- tems that can be housed and operated in basic science laboratories now exist to perform MRI, CT, nuclear imaging, fluorescence and biolumi- nescence imaging on mice (Tab. 1) [7]. In addition, the cost of these sys- tems is generally less than their clinical counterparts and they are thus likely to become routine tools in the development and assessment of new drugs. It has become possible recently to combine imaging modalities, and thus exploit the advantages of two techniques. Examples of such combined sys- tems include CT-SPECT imagers, and the combination of MRI with PET and NIRF imaging. Advances in hardware design and image processing have also been accompanied by advances in probe chemistry and design. Many of the recently developed molecular probes are dual modality and thus capable of being detected by these complimentary imaging techniques [7, 8]. A more detailed description of specific imaging modalities and probe designs follows below. The reader is also referred to a number of excellent recent review arti- cles in this area [5, 9–14]. 2 General approach to molecular imaging The characterization of the human genome has led to an intense focus on genetic models of disease. Although theoretically attractive to image, genetic materials such as DNA and RNA generally do not occur in high enough levels to permit robust imaging. The products of these genetic mate- rials, namely the proteins they encode for, however, do exist in large enough quantities to be imaged. Even so, imaging of proteins whether membrane receptors or enzymes, usually requires an amplification strategy to gener- ate an adequate signal. Amplification strategies may be biological, such as the internalization of a nanoparticle after ligand binding to a particular membrane receptor, or chemical such as the used of biotinylated probes. Alternatively reporter probes may be used to indicate the presence of a pri- mary phenomenon with which they are associated. Examples include the use of thymidine kinase to detect gene expression or cell viability by SPECT 86 Table 1. Overview of high-resolution small-animal imaging systemsa Technique Resolution Depth Time Imaging agents Sensitivity Target Costb Primary small- Clinical animal use use MR imaging 10–100 No limit Min – Gadolinium, dys- ~ 10–6 M A, P, M $$$ Versatile imaging Yes µ m hours prosium, iron modality with high Magnetic resonanceandfluorescencebasedmolecularimagingtechnologies oxide particles soft tissue contrast CT imaging 50 µm No limit Min Iodine ~ 10–3 M A, P $$ Primarily for lung and Yes bone imaging Ultrasound 50 µm Several Min Microbubbles Single A, P $$ Vascular and inter- Yes imaging cm microbubble ventional imaging PET imaging 1–2 mm No limit Min 18F, 11C, 15O~ 10–12 M P, M $$$ Versatile imaging modality Yes with many different tracers SPECT imaging 1–2 mm No limit Min 99mTc, 111In ~ 10–10 M P, M $$ Commonly used to Yes chelates image labeled antibodies, peptides, etc. Fluorescence 2–3 mm <1 cm Sec – Photoproteins ~ 10–10 M P, M $ Rapid screening of Develop- reflectance min (GFP) molecular events in ment imaging (FRI) NIR fluorochromes surface-based tumors Fluorescence 1 mm <10 cm Sec – NIR fluorochromes ~ 10–10 M P, M $$ Quantitative imaging of Develop- mediated min targeted or ‘smart’ fluoro- ment tomography chrome reporters in deep (FMT) tumors Biolumines- Several mm cm Min Luciferins ~ 10–11– M $$ Gene expression, cell and No cence imaging 10–12 M bacterial tracking No Intravital micro- 1 µm <400 µm Sec – Photoproteins ~ 10–10 M P, M $$$ All of the above at higher Limited scopy (confocal, min (GFP) resolutions but at limited develop- multiphoton) fluorochromes depths and coverage ment (skin) a 87 Primary area that a given imaging modality interrogates: A, anatomic; P, physiological; M, molecular targets. Reproduced with permission from [6]. bCost of system: $ <100 K; $$ 100–300 K; $$$: >300 K. David Sosnovik and Ralph Weissleder imaging, and the use of the transferrin receptor as a reporter gene for MR imaging [15–17]. The performance of an imaging modality may be described by its spatial resolution, signal-to-noise ratio (SNR), contrast-to-noise ratio (CNR), and in the case of dynamic phenomena by its temporal resolution as well. Tissue contrast may be generated by intrinsic differences in the properties of tissues, or may require the administration of an imaging agent or reporter probe. The vast majority of molecular imaging techniques have required the develop- ment of probes sensitive to a specific molecular target. The overall perfor- mance of the technique is thus determined by a combination of the charac- teristics of the target, as well as those of the probe and the imaging modal- ity. For instance, the sensitivity of a technique will be influenced by the density and location of the target of interest, the pharmacokinetics of the probe, the vascular and cell membrane permeability of the probe, the affin- ity of the probe for the desired target, and the physical performance of the imaging modality. Probes that alter their physical characteristics upon contacting a desired target have recently been developed and are often described as 'activatable'. Examples of such activatable probes include NIRF agents and smart magnetic relaxation switches [18–20]. Activatable NIRF agents exist in a baseline quenched state until cleavage or activation by the desired enzyme produces fluorescence. The relaxation properties of smart magnetic relaxation switches are likewise changed through an interaction with a specific target. In some ways, these probes can therefore be considered pro-drugs whose final prod- uct produces an imaging readout. Randomized clinical studies have become the ultimate standard for eval- uating the efficacy of a new drug or therapy. However, to achieve statistically significant results, particularly when hard clinical end-points such as myocar- dial infarction or death are used, anywhere from hundreds to tens of thou- sands of patients need to be enrolled in a study. While it is unlikely that the use of molecular imaging will ever make the need for such trials obsolete, molecular imaging has the potential to rationalize the process, reduce the cost of new drug development and ultimately accelerate the introduction of new drugs into clinical practice [6]. 88 Magnetic resonance and fluorescence based molecular imaging technologies 3 Magnetic resonance imaging 3.1
Details
-
File Typepdf
-
Upload Time-
-
Content LanguagesEnglish
-
Upload UserAnonymous/Not logged-in
-
File Pages32 Page
-
File Size-