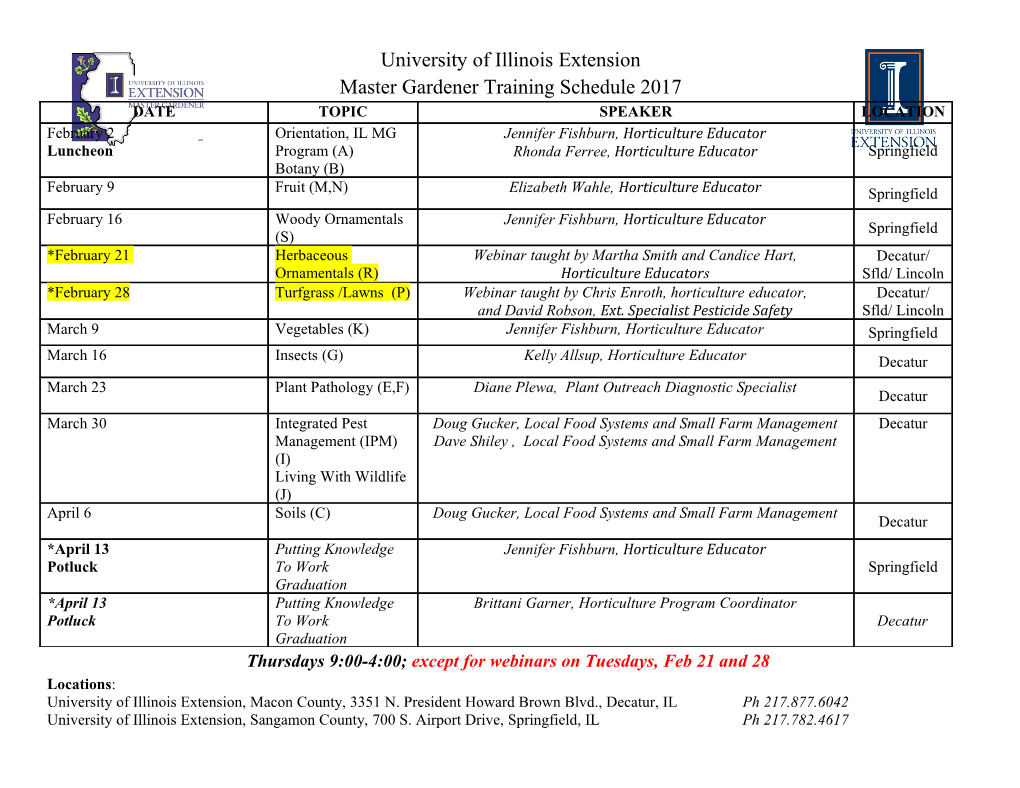
International Journal of Mathematics and M Computer Science, 7(2012), no. 1, 11–83 CS Some infinite series involving the Riemann zeta function Donal F. Connon Elmhurst Dundle Road Matfield, Kent TN12 7HD, United Kingdom email: [email protected] (Received May 16, 2010, Accepted July 12, 2012) Abstract This paper considers some infinite series involving the Riemann zeta function. Some examples are set out below ∞ n n − kxk 1 ( 1) s x, s ,y − x x, s, y n k k y s = Φ( +1 ) log Φ( ) n=0 +1k=0 ( + ) ∞ n n k − n 2 1 − 1−s ζ s ( 1) k k s = 1 2 ( ) n=0 k=0 ( +1) 2 ∞ n n − k − k 1 ( 1) ( 1) π πy n+1 k k y + k − y = cosec( ) n=0 2 k=0 + +1 ∞ 2n 2n u n −1 2 sin 2 u2 u u u u − ζ n3 n =4 log(2 sin )+2Cl3(2 )+4 Cl2(2 ) 2 (3) n=1 ∞ 4 7 1 24n [n!] ζ(3) − πG = n 3 n 2 4 2 n=0 (2 +1) [(2 )!] where Φ(x, s, y) is the Hurwitz-Lerch zeta function and Cln(t) are the Clausen functions. Key words and phrases: Infinite series, Riemann zeta Function. AMS (MOS) Subject Classifications: 40A05, 11M32. 12 Donal F. Connon 1 Some Hasse-type series It was shown in [21] that ∞ n k k y 1 n (−1) y log y = Lis(y) − Lis−1(y)(1) − k s−1 − s 1 n=0 n +1 k=0 (k +1) s 1 where Lis(y) is the polylogarithm function [39] ∞ yn Lis(y)= s n=1 n and, with y = 1, this becomes the formula originally discovered by Hasse [32] in 1930 ∞ n k 1 1 n (−1) = Lis(1) = ζ(s)(2) − k s−1 s 1 n=0 s +1 k=0 (k +1) ∞ s−1 −t The gamma function is defined as Γ(s)= 0 t e dt, s>0. Using the substitution t =(k + y)u,weobtain ∞ 1 1 s−1 −(k+y)u s = u e du (3) (k + y) Γ(s) 0 We now consider the finite sum set out below n k n x Sn(x, y)= (4) k s k=0 (k + y) and combine (3) and (4) to obtain n k n ∞ n x n k 1 s−1 −(k+y)u Sn(x, y)= = x u e du k s k k=0 (k + y) k=0 Γ(s) 0 ∞ n 1 s−1 n k −(k+y)u = u x e du k Γ(s) 0 k=0 Some infinite series involving the Riemann zeta function 13 ∞ n 1 s−1 −yu n −u k = u e xe du k Γ(s) 0 k=0 Using the binomial theorem this becomes ∞ 1 s−1 −yu −u n Sn(x, y)= u e 1+xe du (5) Γ(s) 0 Making the summation, we see that ∞ n k ∞ ∞ n n x 1 n s−2 −yu −u n t = t u e 1+xe du k s n=0 k=0 (k + y) Γ(s) n=0 0 The geometric series gives us for |t (1 + xe−u)|< 1 ∞ n 1 t xe−u 1+ = − −u n=0 1 t (1 + xe ) and we then have ∞ n k ∞ s−1 −yu n n x 1 u e t = du (6) k s − −u n=0 k=0 (k + y) Γ(s) 0 1 t (1 + xe ) We now integrate (6) with respect to t ∞ n+1 n k w ∞ s−1 −yu w n x 1 u e = dt du k s − −u n=0 n +1 k=0 (k + y) Γ(s) 0 0 1 t(1 + xe ) ∞ s−1 −yu − −u − 1 u e log [1 w(1 + xe )] = −u du Γ(s) 0 1+xe and obtain 14 Donal F. Connon ∞ n+1 n k ∞ s−1 −(y−1)u −u w n x 1 u e log [1 − w(1 + xe )] = − du k s u n=0 n +1 k=0 (k + y) Γ(s) 0 e + x (7) When w =1andx →−x we get ∞ n k k ∞ s−1 −(y−1)u −u 1 n (−1) x 1 u e log [xe ] = − du (8) k s u − n=0 n +1 k=0 (k + y) Γ(s) 0 e x ∞ s −(y−1)u ∞ s−1 −(y−1)u 1 u e − log x u e = u du u du Γ(s) 0 e − x Γ(s) 0 e − x We see from (3) that k k ∞ x x s−1 −(k+y)u s = u e du (k + y) Γ(s) 0 and we have the summation ∞ k ∞ ∞ x 1 s−1 −yu k −ku s = u e x e du k=0 (k + y) Γ(s) 0 k=0 1 ∞ us−1e−yu = −u du Γ(s) 0 1 − xe We therefore obtain the well-known formula [43, p. 121] for the Hurwitz- Lerch zeta function Φ(x, s, y) ∞ xk 1 ∞ us−1e−(y−1)u x, s, y du Φ( )= s = u − (9) k=0 (k + y) Γ(s) 0 e x and with y =1weobtain ∞ k ∞ k x 1 x Lis(x) Φ(x, s, 1) = s = s = k=0 (k +1) x k=1 k x Some infinite series involving the Riemann zeta function 15 giving us [46, p. 280] x ∞ us−1 Lis(x)= u du (10) Γ(s) 0 e − x Reference to (8) then shows that ∞ n k k 1 n (−1) x = s Φ(x, s +1,y) − log x Φ(x, s, y) (11) k s n=0 n +1 k=0 (k + y) With x = 1 we obtain Hasse’s formula (2) ∞ n k 1 n (−1) = s Φ(1,s+1,y)=sζ(s +1,y) k s n=0 n +1 k=0 (k + y) With y = 1 equation (11) becomes ∞ n k k 1 n (−1) x = s Φ(x, s +1, 1) − log x Φ(x, s, 1) k s n=0 n +1 k=0 (k +1) or equivalently ∞ n k k 1 n (−1) x s 1 = Lis+1(x) − log xLis(x) (12) k s n=0 n +1 k=0 (k +1) x x which corresponds with (1). A closed form expression may be obtained for example with x =1/2ands =2. We see from (6) that ∞ n k ∞ s−1 −yu n n x 1 u e t = du k s − − −u − n=0 k=0 (k + y) (1 t)Γ(s) 0 1 e tx/(1 t) 1 ∞ us−1e−(y−1)u = u du (1 − t)Γ(s) 0 e − tx/(1 − t) 16 Donal F. Connon and hence referring to (9) we have ∞ n k n n x 1 tx t = Φ ,s,y (13) k s − − n=0 k=0 (k + y) 1 t 1 t With t =1/2weobtain ∞ n k 1 n x =Φ(x, s, y) (14) n+1 k s n=0 2 k=0 (k + y) which is clearly an example of Euler’s transformation of series [33, p. 244]. tx Letting w = 1−t , (13) may be represented by ∞ n n k w n x w + x = Φ(w, s, y) (15) k s n=0 w + x k=0 (k + y) x and with x = −1 this becomes ∞ n n k −w n (−1) =(1− w)Φ (w, s, y) (16) − k s n=0 1 w k=0 (k + y) as previously reported by Guillera and Sondow [57]. Letting w =1andx = −1 in (15) results in another identity due to Hasse ∞ n k 1 n (−1) =Φ(−1,s,y)=ζa (s, y) (17) n+1 k s n=0 2 k=0 (k + y) where ζa (s, y) is the alternating Hurwitz-Lerch zeta function ∞ (−1)k ζa (s, y)= s k=0 (k + y) With y =1wehave ∞ n k 1 n (−1) = ζa (s, 1) = ζa (s) (18) n+1 k s n=0 2 k=0 (k +1) Some infinite series involving the Riemann zeta function 17 Letting w = x = 1 in (15) results in ∞ n 1 n 1 =Φ(1,s,y)=ζ (s, y) (19) n+1 k s n=0 2 k=0 (k + y) Letting s =1andy =1/2 in (17) results in ∞ n k 1 n (−1) = ζa (1, 1/2) n k n=0 2 k=0 2k +1 We have from [15, p. 523] π ζa (1, 1/2) = 2 and we therefore obtain ∞ n k 1 n (−1) π = n k n=0 2 k=0 2k +1 2 which was also determined in a different manner in equation (8.11e) in [22]. Since [15, p. 523] ζa (1,y)+ζa (1, 1 − y)=π cosec(πy) we have for 0 <y<1 ∞ n k k 1 n (−1) (−1) + = π cosec(πy) n+1 k − n=0 2 k=0 k + y k +1 y We now divide (7) by w and integrate to obtain ∞ n+1 n k v −u ∞ s−2 −(y−1)u v n x 1 log [1 − w(1 + xe )] u e = − dw du 2 k s u n=0 (n +1) k=0 (k + y) Γ(s) 0 w 0 e + x Since 18 Donal F. Connon log(1 − ax) dx = −Li2(ax) x we have ∞ n+1 n k ∞ s−1 −(y−1)u −u v n x 1 u e Li2 [v(1 + xe )] = du 2 k s u n=0 (n +1) k=0 (k + y) Γ(s) 0 e + x (20) Further operations of the same kind will result in ∞ n+1 n k ∞ s−1 −(y−1)u −u v n x 1 u e Lir [v(1 + xe )] = du r k s u n=0 (n +1) k=0 (k + y) Γ(s) 0 e + x (21) With t = −1 in (6) we obtain ∞ n k ∞ s−1 −yu n n x 1 u e (−1) = du k s −u n=0 k=0 (k + y) Γ(s) 0 2+xe and letting x →−x we get ∞ n k k ∞ s−1 −yu n n (−1) x 1 u e (−1) = du k s − −u n=0 k=0 (k + y) Γ(s) 0 2 xe With x =2wehave ∞ n k k ∞ s−1 −yu ∞ s−1 −(y−1)u n n (−1) 2 1 u e 1 u e (−1) = du = du k s − −u u − n=0 k=0 (k + y) 2Γ(s) 0 1 e 2Γ(s) 0 e 1 Hence we obtain using (9) ∞ n k k n n (−1) 2 1 (−1) = Φ(1,s,y) (22) k s n=0 k=0 (k + y) 2 Some infinite series involving the Riemann zeta function 19 and with s = n +1andy = 1 this becomes ∞ n k k n n (−1) 2 1 (−1) = ζ(n + 1) (23) k n+1 n=0 k=0 (k +1) 2 This identity, in the case where n is a positive integer, was recently reported by Alzer and Koumandos [6] where it was derived in a very different manner.
Details
-
File Typepdf
-
Upload Time-
-
Content LanguagesEnglish
-
Upload UserAnonymous/Not logged-in
-
File Pages73 Page
-
File Size-