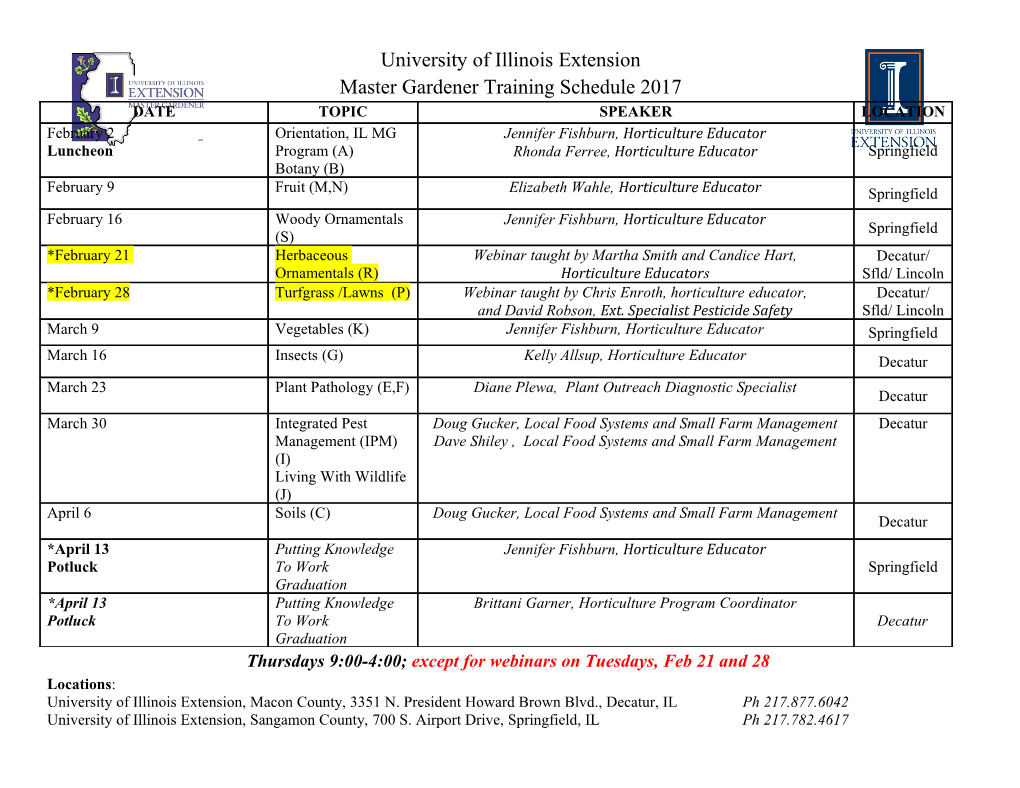
HydroGIS 96: Application of Geographic Information Systems in Hydrology and Water Resources Management (Proceedings of the Vienna Conference, April 1996). IAHSPubl. no. 235, 1996. 469 Application of WEPP and GIS on small watersheds in USA and Austria M. R. SAVABI USDA-ARS, National Soil Erosion Research Laboratory and Agricultural and Biological Engineering Department, Purdue University, West Lafayette, Indiana 47907, USA A. KLIK University of Renewable Resources, Vienna, Austria K. GRULICH Agricultural and Biological Engineering Department, Purdue University, West Lafayette, Indiana 47907, USA J. K. MITCHELL Agricultural Engineering Department, University of Illinois, Urbana, Illinois, USA M. A. NEARING USDA-ARS, National Soil Erosion Research Laboratory, West Lafayette, Indiana 47907, USA Abstract Geographic Information Systems (GIS) are well structured databases for handling large quantities of spatially varied data within a watershed. Coupling of a GIS with a spatially variable, physically-based, deterministic hydrological model such as the USDA-Water Erosion Prediction Project (WEPP) offers many advantages. The WEPP model is a new computer program based on the fundamentals of hydrology, soil physics, plant science, hydraulics, and erosion mechanics. The WEPP model provides several major advantages over existing hydrological and erosion models; for example, it reflects the effects of soil surface conditions due to agricultural, range and forestry practices on storm runoff and erosion. The Geographical Resources Analysis Support System (GRASS) GIS was used to obtain many of the needed input parameters for the WEPP computer model. The results indicate GRASS- GIS technology is a powerful tool and can be used to parameterize a complex hydrological model such as WEPP. INTRODUCTION Computer models that are process-oriented, physically-based, and therefore, mathemati­ cally mimic the spatial behaviour of a hillslope, watershed and/or basin's conditions are effective tools for examining the impact of various management decisions on water resources of a region. However, the amount of data and required parameters increase as the models become more complex. In recent years, GIS has become useful spatial data handling tools. Databases resulting from the combination of distributed information maps can support spatially distributed hydrological models. Several investigators have 470 M. R. Savabi et al. successfully integrated hydrological models with GIS (Mallants & Badji, 1991; Savabi et al., 1995). The common agreement is that GIS is a convenient and well structured database for handling large quantities of spatially varied data for a watershed and entire basin. The objective of this study is to explore the feasibility of using GIS to obtain some of the required parameters to test the WEPP (USDA, 1995) computer model for application under different crop conditions and geographic locations. MATERIALS AND METHODS WEPP model The WEPP computer model can be divided into six conceptual components: climate generation, hydrology, plant growth, soils, management effect, and erosion. A brief description of each component is given here. The meteorological data required by the WEPP model can be generated, if not available, by a separate computer model called CLIGEN (USDA, 1995). Based on long-term statistics from historical climate data, the CLIGEN model generates daily values of required meteorological data for a station near the desired simulation location. Precipitation may be either in the form of rain or snow, depending upon the temperature. Rainfall is disaggregated into a time-rainfall intensity format for use by the infiltration and erosion components. If meteorological data for a location are available, the user can create the climate file using the observed climate and may also enter breakpoint precipitation information. The hydrology component includes simulation of storm runoff, snow melt, soil evaporation, plant transpiration, percolation, irrigation, and subsurface flow (Savabi, 1993). Excess rainfall is calculated as the difference between rainfall rate and infiltration rate. Infiltration rate is calculated using the Green and Ampt equation for unsteady rainfall. The effect of spatial and temporal variability of soil water intake is simulated by adjusting the effective hydraulic conductivity of the soil for human disturbances such as tillage, and natural phenomena such as soil surface sealing and vegetal roots. Excess rainfall is routed down slope to estimate the overland flow hydrograph using a kinematics wave approach (USDA, 1995). Peak runoff and runoff duration are used in calculating flow shear stress, sediment transport capacity, and rill erosion. The WEPP winter component predicts frost and thaw layer development, snow accumulation and snow melt. The subsurface drainage component simulates water flow to subsurface tile drains and/or drainage ditches (Savabi, 1993). The plant growth model in WEPP assumes phenological crop development based on daily accumulated heat units, and a harvest index for partitioning grain yield. Many of the soil parameters used within the WEPP model change with time as a result of field operations, freezing, thawing, and weathering. The soil component simulates the temporal variability of soil properties such as bulk density, hydraulic conductivity, surface roughness, and erodibility parameters (USDA, 1995). The effect of various land management practices on hydrology and erosion for a site can be simulated with the WEPP model. The management component uses the data contained in the management input file to determine changes in soil physical properties and surface roughness and cover conditions due to practices such as tillage, crop harvest, grazing, and various residue management options (USDA, 1995). Application of WEPP and GIS on small watersheds in USA and Austria 471 The erosion component uses a steady-state sediment continuity equation as the basis for the erosion computations. Soil detachment in the interrill areas is calculated as a function of effective rainfall intensity and runoff rate. Soil detachment in the rills is predicted to occur if the flow hydraulic shear stress is greater than critical shear and the flow sediment load is below transport capacity. Deposition in the rills is computed when the sediment load is greater than the capacity of the flow to transport it (USDA, 1995). Model parameters The WEPP hillslope profile erosion model requires a minimum of four input data files: climate, soil, slope, and plant/management. Climate input files include daily precipitation amount, duration of storm, maximum intensity of storm, time to maximum storm intensity, maximum and minimum temperatures, solar radiation, wind speed and direction, and dew point temperature. Soil input files include such soil parameters as albedo, initial soil water content, soil textures, effective hydraulic conductivity, rock content, percent organic matter in the soil, and soil cation exchange capacity (CEC). The slope input file includes physical features such as slope length, slope steepness, and profile aspect. The plant/management file requires land use (agriculture, range, or forest) to be identified by users. For each land use, information about the specific plants present and management practices used are needed. For instance, for cropland, information about the crop plant growth (such as planting and harvest dates), type and dates of tillage, and type and dates of residue management are required. Watershed descriptions Austrian watersheds Three watersheds at Mistelbach, Kuffern and Feldbach were selected for this study. For the Mistelbach and Kuffern watersheds stereo photos with a scale of 1:15 000 were available. From these photos the topography was derived for a grid of 20 m distance. This grid then was used to derive the Digital Elevation Map (DEM) by using the computer driven SCOP method (Molnar, 1992). Because of the small scale of the aerial photos, the accuracy of the DEM is ca. 30 cm. The DEM for Feldbach is based on grid data (with a point distance of 50 m) from the Federal Surveyor's Office. Using the DEM, maps with contour lines, different slope classes and slope vectors were drawn. The maps of flow vectors were used to get the watershed boundaries, the boundaries of the sub watersheds and the lengths of the longest hillslopes of each subwatershed. The watersheds were represented by several hillslopes along the longest runoff pathway (Table 1, Fig. 1). Each hillslope was subdivided into Overland Flow Elements (OFE), depending on soil type and crops. The elaboration of all three watersheds was done by the Institute for Photogrammetry and Remote Sensing at the Technical University of Vienna. The Mistelbach and Kuffern watersheds have similar soil types that are deep, well drained and have a mollic epipedon with textures of loam and silt loam. Most of the soils have, according to the Austrian Soil Survey Reports (Oesterreichische Bodenkartierung, 1995), "moderate" hydraulic conductivity. In Mistelbach, eight different soil types can be classified, while in Kuffern and Feldbach there are only seven and five soil types, 472 M. R. Savabi et al. Table 1 Size, slope, length, mean annual precipitation, and daily maximum precipitation. Mistelbach Kuffern Feldbach Size (ha) 37.46 38.95 31.14 Average slope (%) 7.8 9.6 18.1 Max. length (m) 900 690 1009 Number of hillslopes 6 5 1 Number of OFE per hillslope 10 8 9 Mean annual precipitation (mm) 539 695 744 Daily max. precipitation (100 years, mm) 49
Details
-
File Typepdf
-
Upload Time-
-
Content LanguagesEnglish
-
Upload UserAnonymous/Not logged-in
-
File Pages8 Page
-
File Size-