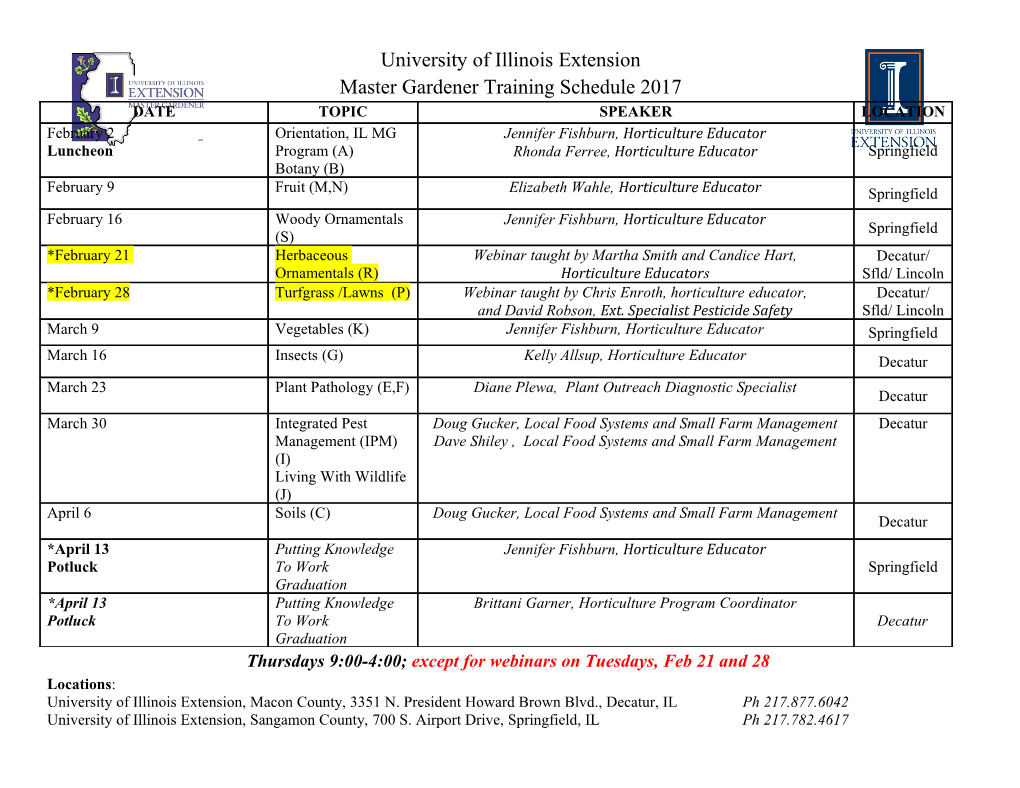
REPRINTED FROM MODELS AND GREAT BASIN PREHISTORY A SYMPOSIUM EDITED BY DON D. FOWLER DESERT RESEARCH INSTITUTE PUBLICATIONS IN THE SOCIAL SCIENCES NO, 12 EDITOR: DON D. FOWLER ASSOCIATE EDITOR: ALMA SMITH 1977 GREAT BASIN LATE QUATERNARY ENVIRONMENTS AND CHRONOLOGY' PETER J. MEHRINGER, JR. WASHINGTON STATE UNIVERSITY ABSTRACT Evidence for the magnitude and chronology of Great Basin climatic change comes from plant macrofossils of dry caves, fluctuating lake levels, spring discharge and tree lines, dune activity, peat formation in desert salt marshes, and arroyo cutting and filling. About 12,000-10,000 B.P. pluvial lakes began to dry and by 7500 B.P. climatic conditions were much like the present. With minor fluctuations, relative aridity per- sisted until about 4000 B.P. and was followed by increased effective moisture. The dynamic nature of Great Basin environments is apparent whether measured by geological or biological criteria. There were several periods when regional climatic change was sufficient to warrant investigations of its possible influence on human populations; locally, volcanic or tectonic activity may have been even more important. How- ever, instability of the last 10,000 years is no more dramatic than the ecological variation encountered by Great Basin inhabitants within a single year. Variability itself may have been most important in shaping cultural or technological adaptations. The influence of climatic change on man is best considered in terms of evidence for its effect on local resources. INTRODUCTION Great Basin anthropologists have assumed an important link between man and environment, and interpretations of cultural change have been influenced by notions of the magnitude and chronology of climatic history. Conversely, varying views on climatic change have been influenced by interpretations of Great Basin cultural history. The relation between climate and culture has been expressed in concern with environmental influences on 113 population density and distribution, resource utilization, and technology (Ranere 1970). Environmental factors have undoubted- ly influenced the course of Great Basin technological and cultural adaptation, just as man's exploitation of natural resources continues to shape the course of civilizations. How- ever, as the smartest (if not always the wisest) and most adaptable of all creatures, man does not usually qualify as a sensitive biological indicator species. Along with a discussion of the application of paleo- ecological models to Great Basin archeology (see Weide and Weide, this volume), it is important to reconsider the primary data from which inferences are drawn, and the geographical and chronological extent of their application to prehistory. Generalized models relating cultural change to synchronous and unidirectional climatic change are simple to construct and easy to use. For example, for more than 20 years the human response to a supposed extreme mid-postglacial drought pro- vided a focus for constructive discussion and unrestrained argument among Great Basin archeologists. However, the inter- pretation of paleoenvironmental data, the question of their correspondence over the entire Great Basin and their meaning to human history become increasingly less certain as informa- tion from specific localities reveals a chronological and geographical complexity few expected when the last 10,000 years could simply be divided into three geologic-climatic stages and the cultural sequences nearly always fit. We should, perhaps, retreat for a time to less encompassing models and explanations. Fortunately, the Great Basin pro- vides a laboratory of unusual variety and potential for studies of environmental change and human response. In this review I will attempt to describe some of what is known of the magnitude and chronology of Great Basin environmental changes and their possible influences on the prehistoric in- habitants. The information reviewed herein is not fitted to an exist- ing climatic chronology or its presumed effect on man (D. Fowler 1972a); that has been done before (Baumhoff and Heizer 1965). Rather, the kinds of data, their interpretation, and possible relation to human occupation are presented both chronologically and regionally. Since man's tenure in North America remains uncertain, the last 40,000 years are included but the last 12,000 are emphasized. For convenience the re- view is artificially separated into geological and biological evidence for environmental change. Geological evidence in- cludes lake level fluctuations, glaciation, volcanic and tectonic activity, soils, sediments and erosion, and eolian activity. Biological evidence includes the fossil records of plants and animals, dendroclimatology, and some biogeographic considerations. All of these may be indicators of past climates or directly affect important resources. 114 4 THE GEOLOGICAL EVIDENCE Pluvial Lakes Among the more important aspects of Great Basin geology is evidence for former moist-cool periods accompanying glaci- ation. While the concept of worldwide "pluvials" everywhere coeval with glaciation is questionable, the gross chrono- logical correspondence between Great Basin lakes and continen- tal glaciers clearly illustrates a related cause in Pleistocene atmospheric circulation over North America. Generally low lake levels from about 40,000-30,000 to 24,000 B.P. and deep lakes from 24,000 to 12,000 B.P. are of possible importance to Great Basin occupation. The evidence for pluvial lakes (Figs. 1,2,3) includes strandlines, algal tufas, deltas, bars, and subsurface stratigraphy and sediments. These obvious features of lake and river systems, common throughout the Great Basin, were studied during early geological and hydrological investi- gations (Gale 1914; Gilbert 1890; Meinzer 1922; Russell 1885). The use of "pluvial" to indicate increased precipitation is perhaps misleading, as the exact climatic parameters res- ponsible for any single lake level are unknown. The Spring Valley, Nevada, studies of Snyder and Langbein (1962) are the most thorough to date and illustrate the improbability of a single cause; both decreased evaporation and increased pre- cipitation were most probably required for maximum lake levels. Morrison's (1965a:267) use of "lacustral" avoids the connota- tions of pluvial. Galloway (1970:256) substitutes "minevaporal" for pluvial to indicate the importance of decreased temperatures. Estimates of climatic change required to account for high lake levels are given in Table 1 and reviewed by Morrison (1965a:281). Table 1 Climatic Change Inferred from Estimates of Western United States Pluvial Lake Water Budgets, as Compared With Historic Mean Annual Climatic Data Temperature Precipitation Reference (deg. F.) (inches) Meinzer 1922 -15 Leopold 1951 -11 to 12 +7 to 9 Antevs 1952 -5 +6 Broecker and Orr 1958 -9 +8 Snyder and Langbein 1962 -9* +8 Galloway 1970 -18 to 20 -0.6 to 1.7** *Estimated from a 30% (13 inch) decrease in evaporation given by Snyder and Langbein. **Calculated from Table 3 of Galloway. 115 LAKE BONNEVILLE OREGON 1 IDAHO NEVADA LAKE LAHONTAN o 0 a er. '0 a 0 04 2 0 ; p UTAH ‘\ . 0 I ° 4d ,, b 4 \v:\ LAKE SEARLES., Ix\S e \ , N MOHAVE (7:9 0 50 100 150 200 250 MILES 0 100 200 300 400 KILOMETERS Fig. 1. Great Basin pluvial lakes (after Snyder, Hardman and Zdenek 1964) 116 Fig. 2. The Great Bar at Stockton, Utah (Gilbert 1890 , plate IX), a gravel bar separating Tooele and Rush Valleys, is one of the more spectacular features of Lake Bonneville. Its wave cut surface is near- ly 1000 feet above the present Great Salt Lake (April 1970). Fig. 3. Barren lake beds and strand lines of Lake Searles (Septem- ber 1967). 117 Four pluvial lakes (Bonneville, Lahontan, Searles, and Mohave) have been studied in considerable detail. The magni- tude and chronologies of their fluctuations illustrate present understanding of Great Basin pluvial lake history (Figs. 4,5). Interpretations of the radiocarbon chronologies vary; one im- portant reason is the differences in the reliability of materials dated. Thus, various interpretations depend on the researchers, the dates they accept, and the materials dated (wood, shell, whole core, core humates, strandline tufa, or subsurface car- bonates). For example, discrepancies are apparent in proposed chronologies for Lakes Lahontan and Bonneville (Fig, 4). Broecker and Kaufman's (1965:549, figs. 4,5,6) suggested very deep lake cycles after about 12,000 B.P. conflict with other interpretations (Born 1970:84; Eardley, Gvosdetsky and Marsell 1957:1169-1171; Morrison 1970; Morrison and Frye 1965:23). I interpret selected evidence (Bright 1966:27; Jennings 1957:97) as indicating that Lake Bonneville did not overflow after about 12,500 B.P., fell rapidly to below the level of Danger Cave (about 4300 feet and less than 100 feet above the highest his- toric stand of Great Salt Lake) by about 11,000 B.P. and did not rise above Danger Cave thereafter. The Searles Lake chronology (Fig. 5), like that of Lahontan and Bonneville, reflects a late Wisconsin period of high water levels following smaller lakes correlated with the preceding interstadial (G. Smith 1967a, 1968, fig. 4; Smith, Friedman and Matsuo 1970). Lake history is recorded in the classic sub- surface stratigraphy with salts representing shallow lakes and muds representing deep lakes. Searles Lake received drainage from the Sierra Nevada via the Owens River, Owens Lake, and China Lake and overflowed into Panamint Valley which in turn overflowed to Death Valley, the ultimate pluvial-maximum sink for the Mohave and Amargosa rivers as well. , Because of its position in a long chain of lakes, the precise chronology of the last major Lake Searles overflow is critical to understand- ing the climatic and biographic history (Miller 1950) of the Death Valley system. Hooke (1972:2093) assumes that lakes in Death Valley fluctuated in phase with Lake Searles and, further, that Pana- mint Valley most probably overflowed into Death Valley via Wingate Wash between 11,000 and 10,500 B.P. A shallow marsh deposit on the floor of North Panamint Valley is radiocarbon dated at 10,000-10,500 B.P. (Davis, Brott and Weide 1969:15; Mehringer 1967a:172, table 5).
Details
-
File Typepdf
-
Upload Time-
-
Content LanguagesEnglish
-
Upload UserAnonymous/Not logged-in
-
File Pages58 Page
-
File Size-