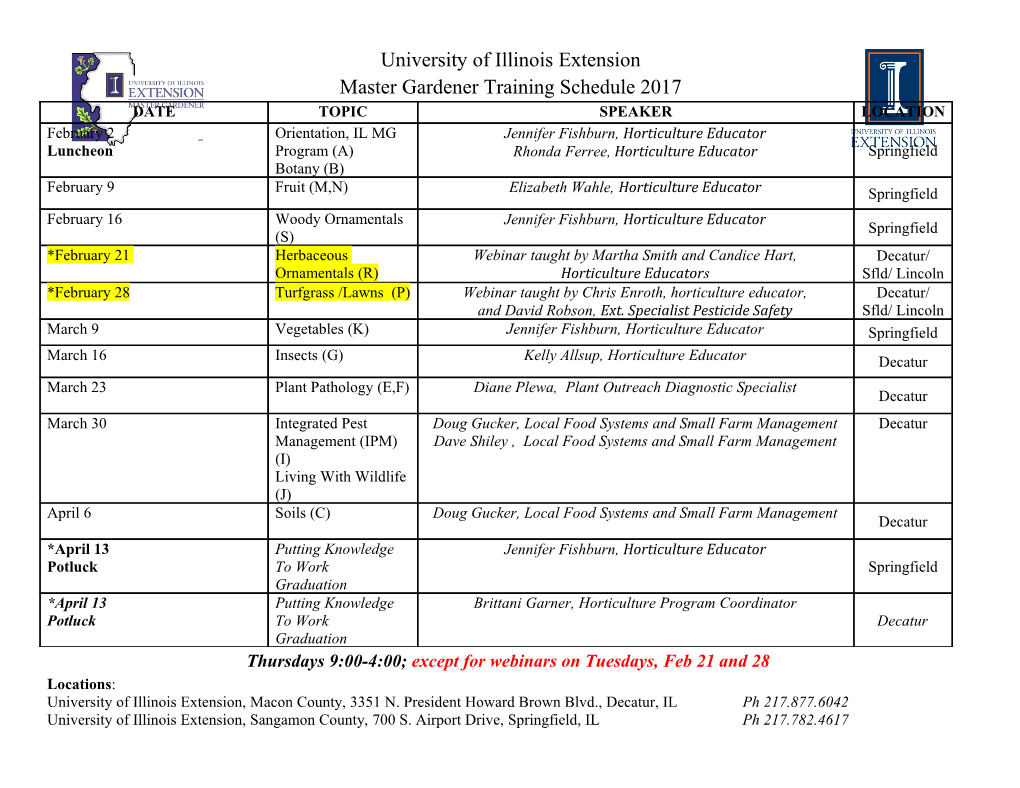
Cancellation of infrared divergences in QCD Daniel Wennberg Physics specalization project Submission date: January 2014 Supervisor: Michael Kachelrieß Norwegian University of Science and Technology Department of Physics Abstract The scattering process hadrons is considered at next-to- + − leading order, . It is demonstrated푒 푒 → that the cross sections for both the real gluon emission풪(훼푠) and virtual gluon exchange have infrared singu- larities. Using dimensional regularization it is shown that the divergent terms cancel exactly when adding the contributions, such that the total cross section for hadrons is finite at this order. This result, and + − the general implications푒 푒 → of infrared divergences and their cancellation, is discussed in light of the Kinoshita-Lee-Nauenberg theorem and the concept of infrared safety. 2 Contents List of figures 5 List of tables 6 List of symbols and notation 7 Preface 9 1 Introduction 10 1.1 Perturbative quantum chromodynamics . 10 1.2 Infrared divergences . 13 2 Cancellation of infrared divergences in hadrons 14 + − 2.1 Leading order amplitude for 푒 푒 →. 15 + − 2.2 Cross sections for hadrons푒 푒 → . 푞 .푞 . 19 + − 2.3 Real gluon emission:푒 푒 → at leading order . 21 + − 2.4 Virtual gluon exchange:푒 푒 → 푞푞푔 at next-to-leading order . 26 + − 2.5 Cancellation: hadrons푒 푒 → at 푞 next-to-leading푞 order . 34 + − 2.6 The ultraviolet푒 divergence푒 → . 35 3 Discussion 38 3.1 Infrared divergences in general quantum field theories . 38 3.2 The optical theorem and cutting rules . 41 3.3 Infrared safety in QCD . 44 4 Summary 46 A Feynman rules for QED and QCD 47 A.1 General remarks . 47 A.2 QED . 49 A.3 QCD . 51 A.4 Polarization . 52 A.5 Some results from ..................... 53 SU(푁푐) 3 Contents B Properties of gamma matrices and Dirac spinors 56 B.1 Gamma matrices . 56 B.2 Dirac spinors . 60 C Dimensional regularization 66 C.1 Important identities . 68 C.2 Phase space in dimensions . 69 푑 Bibliography 72 4 List of figures 2.1 Leading order diagram for . 16 − + 2.2 Leading order diagrams for푒 푒 → 푞푞 . 22 − + 2.3 Diagrams for at one푒 푒 loop→ 푞.푞푔 . 27 − + 푒 푒 → 푞푞 3.1 Forward scattering .................... 42 + − + − 3.2 Cut diagrams for 푒 푒 hadrons→ 푒 푒 . 43 ∗ 훾 → A.1 Example of a Feynman diagram . 47 5 List of tables 1.1 Masses of quarks and leptons . 12 A.1 Building blocks of QED Feynman diagrams . 50 A.2 Lines in QCD Feynman diagrams . 51 A.3 Vertices in QCD Feynman diagrams . 52 6 List of symbols and notation The coupling constant of quantum chromodynamics; . 2 푔푠, 훼푠 훼푠 ≡ 푔푠 /4휋 em The positive elementary electric charge and coupling constant of quan- 푒, 훼 tum electrodynamics; em . The electron charge is . 2 훼 ≡ 푒 /4휋 −푒 The electric quantum number of a quark ; the quark charge is . 푒푞 푞 푒푒푞 The complex conjugate of the number : . Applies ∗ ∗ 푧 elementwise to vectors, matrices etc. 푧 = 푥 + 푖푦 푧 = 푥 − 푖푦 The real part of the complex number : . ∗ ℜ{푧} 푧 ℜ{푧} = (푧 + 푧 )/2 The imaginary part of the complex number : . ∗ ℑ{푧} 푧 ℑ{푧} = (푧 − 푧 )/2푖 The identity matrix. 푰 The transpose of the matrix : . 푇 푇 퐴 퐴 (퐴 )푖푗 = (퐴)푗푖 The Hermitian adjoint of the matrix : . † † ∗ 푇 퐴 퐴 퐴 = (퐴 ) The metric tensor in Minkowski space, with signature . 휇휈 휂 (+, −, −, −) The spatial part of a 4-vector : . 0 0 1 2 3 1 2 3 풂 푎 = (푎 , 풂) = (푎 , 푎 , 푎 , 푎 ) 풂 = (푎 , 푎 , 푎 ) The scalar product of two spatial vectors: . 푖 푖 풂 ⋅ 풃 풂 ⋅ 풃 = 푎 푏 The scalar product of a spatial vector with itself: . 2 2 풂 풂 = 풂 ⋅ 풂 The length of a spatial vector: . Also used for Euclidean lengths 2 ̃푎 of vectors in arbitrary dimensions.̃푎= √풂 The inner product of two 4-vectors: . 휇 0 0 푎 ⋅ 푏 푎 ⋅ 푏 = 푎 푏휇 = 푎 푏 − 풂 ⋅ 풃 The inner product of a 4-vector with itself: . 2 2 휇 0 2 2 푎 푎 = 푎⋅푎 = 푎 푎휇 = (푎 ) −풂 The energy fraction of particle in the COM frame: with 2 푥푖 . 푖 푥푖 = 2푝푖 ⋅ 푄/푄 푄 = ∑푖 푝푖 7 List of symbols and notation The Dirac gamma matrices. 휇 훾 The Dirac adjoint of a Dirac spinor . † 0 휓 휓 = 휓 훾 휓 The Feynman slash, denoting the contraction of a 4-vector and the 푎 gamma matrices: . / 휇 /푎 = 푎휇훾 Dirac spinor for a fermion with spin state and momentum . 푠 푢 (푝) 푠 푝 Dirac spinor for an antifermion with spin state and momentum . 푠 푣 (푝) 푠 푝 A component of a 4-vector , specified by a greek index 휇 0 1 2 3 푎 , known as a푎 Lorentz = (푎 , 푎 index., 푎 , 푎 ) The index may be lowered 휇by ∈ contraction {0, 1, 2, 3} with the metric tensor: . 휈 푎휇 = 휂휇휈푎 A spatial component of a 4-vector , specified by a latin 푖 0 1 2 3 푎 index . 푎 = (푎 , 푎 , 푎 , 푎 ) 푖 ∈ {1, 2, 3} The number of color degrees of freedom for quarks. For real-world 푁푐 quarks, . 푁푐 = 3 The Gell-Mann matrices, listed in equation (A.15). 푎 휆 The generators of the fundamental representation of . For 푎 푇 defined as SU(푁푐) 푁푐 = 푎 푎 3 푇 = 휆 /2 The structure constants of the generator algebra: . 푎푏푐 푎 푏 푎푏푐 푐 푓 [푇 , 푇 ] = 푖 푓 푇 The Dynkin index of the generator algebra: . For 푎 푏† 푎푏 푇퐹 and , . Tr [푇 푇 ] = 푇퐹훿 푎 푎 푁푐 = 3 푇 = 휆 /2 푇퐹 = 1/2 The color factor of the generator algebra: . For 푎 푎† 퐶퐹 and , . (푇 푇 )푖푘 = 퐶퐹훿푖푘 푁푐 = 3 푎 푎 푇 = 휆 /2 퐶퐹 = 4/3 The Feynman propagator for a gauge boson with momentum . 휇휈 퐷퐹 (푝) 푝 The Feynman propagator for a fermion with momentum . 푆퐹(푝) 푝 A general scattering amplitude. ℳ The average over initial spin and color states and sum over final spin 2 ∣ℳ∣ and color states of . 2 ∣ℳ∣ The Einstein summation convention applies to all kinds of indices unless otherwise stated, e.g. For Lorentz indices, there must always be one upper and one lower푎푖푏푖 = summation ∑푖 푎푖푏푖 index: . 휇 휇 휇 휈 Units are chosen such that . 푎 푎휇 = ∑휇 푎 푎휇 = ∑휇휈 휂휇휈푎 푎 ℏ = 푐 = 1 8 Preface This report is the outcome of my work in the physics specialization project, TFY4510, carried out during the fall semester of 2014 under supervision of Prof. Michael Kachelrieß at the Department of Physics at the Norwegian University of Science and Technology (NTNU). The objective of the project has been to learn about the phenomenon of infrared divergences and how they cancel when defining observables satisfying particular requirements, mainly in the context of quantum chromodynamics and with electron-positron annihilation to quarks as the process of study. Infrared divergences have not been treated in detail during the course of my studies, and the topic was therefore largely unknown to me before embarking on this project. The main motivation for studying this is to prepare for a possible Master’s thesis studying annihilations in a wino-like Dark Matter candidate model. As the project work has consisted solely of gaining acquaintance with the subject matter, there are no new results to present. The report therefore amounts to a thorough presentation of established theoretical results, reflecting my level of knowledge as of finishing this project. The aim has been to give a comprehensive and largely self-contained presentation of the main ideas and derivations, including a significant amount of background material that I had to learn or review in order to get through the calculations. Much of this has been relegated to appendices in an attempt to obtain a readable and reasonably well-structured text, but I still consider it an integral part of my project work. 9 1 Introduction Quantum chromodynamics (QCD) is the theory describing the strong interac- tions between the quarks and gluons, the fundamental particles which make up baryons such as the proton and neutron, and mesons such as the pion. The quarks and gluons carry a quantum number which is specific to QCD, known as color charge. QCD is a quantum field theory described by the Lagrangian [1, pp. 21-22] (1.1) 푎 푎 1 푎휇휈 푎 ℒQCD = 휓푞,푖 (푖휕/ − 푚푞) 훿푖푗휓푞,푗 − 푔푠휓푞,푖푇푖푗퐴/ 휓푞,푗 − 퐹 퐹휇휈 , Here, is the Dirac spinor field for a quark flavor labeled4 , with mass , while 휓푞 is the field strength tensor for the gauge field 푞 describing푚 the푞 푎 푎 gluons.퐹휇휈 The indices label the color charge basis퐴휇 states of the quarks, while the index푖, 푗 ∈ {1, 2, 3} labels color charge basis states for the gluons. The terms are further푎 ∈ {1, discussed … , 8} in appendix A. Quarks also carry electric charge and are subject to interactions in quantum electrodynamics. These interactions are not present in equation (1.1) but are discussed in appendix A.2. Finally, quarks interact weakly, but weak interactions will not be discussed in this text. 1.1 Perturbative quantum chromodynamics When applying the usual tools of perturbative quantum field theory to the QCD Lagrangian, the Feynman rules in appendix A.3 appear. Compared to for example quantum electrodynamics (QED), there is however a significant complication when predicting experimental outcomes using this formalism: perturbative quantum field theory describes scattering processes where the initial and final states consist of free particles in the fundamental fields in the Lagrangian, but free quarks or gluons are never observed in experiments.
Details
-
File Typepdf
-
Upload Time-
-
Content LanguagesEnglish
-
Upload UserAnonymous/Not logged-in
-
File Pages73 Page
-
File Size-