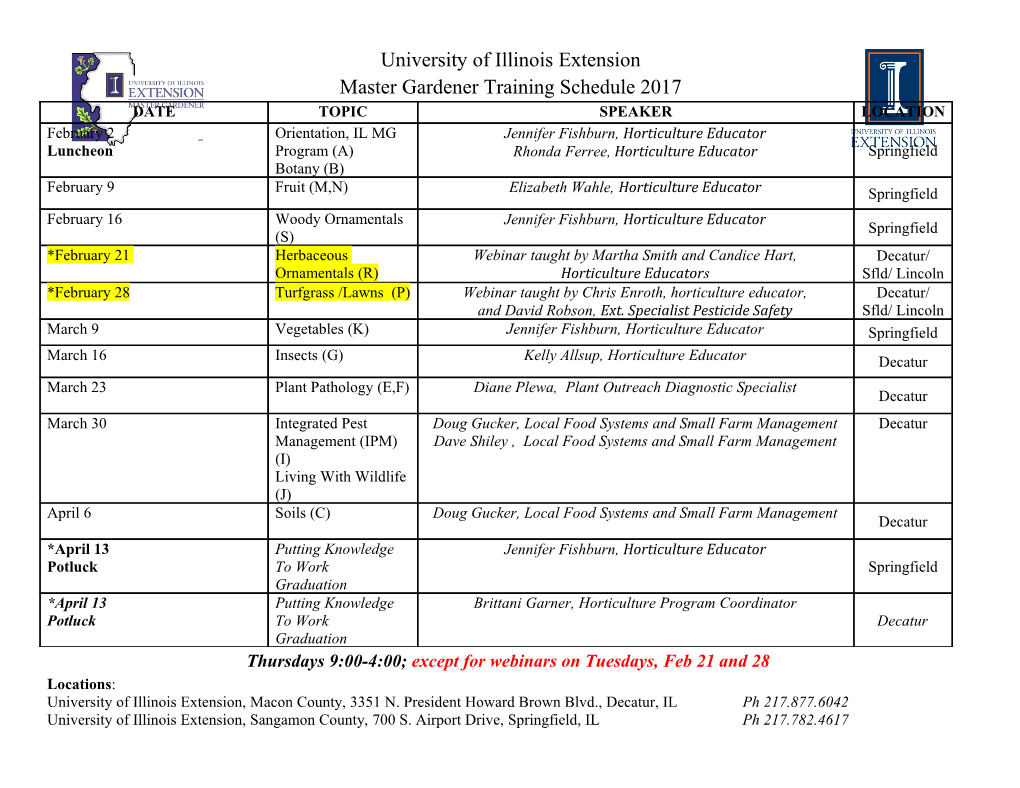
Advances in Colloid and Interface Science 232 (2016) 80–100 Contents lists available at ScienceDirect Advances in Colloid and Interface Science journal homepage: www.elsevier.com/locate/cis Historical perspective The polymer physics of single DNA confined in nanochannels Liang Dai a, C. Benjamin Renner b, Patrick S. Doyle a,b,⁎ a BioSystems and Micromechanics (BioSyM) IRG, Singapore-MIT Alliance for Research and Technology (SMART) Centre, 138602, Singapore b Department of Chemical Engineering, Massachusetts Institute of Technology (MIT), Cambridge, MA 02139, United States article info abstract Available online 23 December 2015 In recent years, applications and experimental studies of DNA in nanochannels have stimulated the investigation of the polymer physics of DNA in confinement. Recent advances in the physics of confined polymers, using DNA Keywords: as a model polymer, have moved beyond the classic Odijk theory for the strong confinement, and the classic blob Polymer physics theory for the weak confinement. In this review, we present the current understanding of the behaviors of DNA confined polymers while briefly reviewing classic theories. Three aspects of confined DNA are presented: static, Nanochannel dynamic, and topological properties. The relevant simulation methods are also summarized. In addition, compar- Confinement isons of confined DNA with DNA under tension and DNA in semidilute solution are made to emphasize universal Monte Carlo simulation behaviors. Finally, an outlook of the possible future research for confined DNA is given. © 2015 Elsevier B.V. All rights reserved. Contents 1. Introduction...............................................................81 2. DNAmodelandsimulationmethod.....................................................82 2.1. Touching-beadmodel........................................................82 2.2. Freely-jointedrodmodel......................................................83 2.3. Otherpolymermodels.......................................................84 2.4. MetropolisMonteCarlosimulation..................................................84 2.5. SimplifiedPERMsimulation.....................................................84 2.6. Comparisonofsimulationmethods..................................................85 2.7. Othersimulationmethods......................................................85 3. Polymer physics of confinedDNA......................................................85 3.1. Static properties of confinedDNA..................................................85 3.1.1. ClassicdeGennesregime..................................................86 3.1.2. ExtendeddeGennesregime.................................................87 3.1.3. ClassicOdijkregime....................................................88 3.1.4. BackfoldedOdijkregime..................................................89 3.2. Dynamic properties of confinedDNA.................................................92 3.2.1. Dynamicsofasinglepolymerinbulk.............................................92 3.2.2. Hydrodynamicinteractioninfreespace...........................................92 3.2.3. Hydrodynamic interaction in confinement..........................................94 3.2.4. Diffusivity of confinedDNAinslits..............................................94 3.2.5. Relaxation of confinedDNA.................................................96 3.3. Topological properties of confinedDNA................................................96 3.3.1. DNAknotsinfreespace...................................................97 3.3.2. DNAknotsinnanochannels.................................................97 4. Comparison of confinedpolymersandpolymerunderotherconditions.....................................97 4.1. Polymers in confinementversuspolymersundertension........................................98 4.2. Polymers in confinementversuspolymersinsemidilutesolution....................................98 ⁎ Corresponding author. E-mail address: [email protected] (P.S. Doyle). http://dx.doi.org/10.1016/j.cis.2015.12.002 0001-8686/© 2015 Elsevier B.V. All rights reserved. L. Dai et al. / Advances in Colloid and Interface Science 232 (2016) 80–100 81 5. Summaryandoutlook........................................................... 98 Acknowledgment............................................................... 98 References.................................................................. 98 1. Introduction In recent years, experiments on DNA in nanochannels [1–7] and their relevant applications [8–14] have stimulated the systematic study of the physics of confined DNA molecules. These experiments have been made possible due to concurrent advances in nanofabrication techniques [15–27]. Beyond practical applications, DNA has often been used as a model semiflexible polymer for single-molecule experiments for the purpose of exploring general polymer physics under confine- ment [28]. Single DNA molecules with well-defined length can be pre- pared by the molecular biology techniques, and visualization of single DNA molecules is convenient with the aid of various fluorescence dyes. Two examples [2,29] of the visualization of single DNA molecules in two types of confinement: tube-like channels [2], and the slit-like channels [29] are shown in Fig. 1. One promising application of confining DNA in nanochannels is ge- nome mapping [9,30].Thebasicpremise,showninFig 2, involves label- ing specific sequence motifs in DNA molecules by fluorescence dyes, confining and stretching these molecules in nanochannels, and then in- ferring the number of base pairs between sequence motifs by measuring the distances or the fluorescence intensities between motifs. The sequence motif map can be used directly or facilitate the assembly of short DNA sequences [30]. Many experiments [14,24,31] and simula- tions [32] have been performed towards the development of this tech- nological platform. Confining DNA in nanochannels has been also applied for other applications, such as DNA sorting [26,33,34],DNA denaturation mapping [11,35], recognizing barcoded DNA [12],and studying DNA-protein interactions [36]. From the viewpoint of polymer physics, confinement is a type of perturbation to polymer systems, providing many fundamental ques- tions to be answered. Intuitively, confining a DNA molecule within a nanochannel will elongate the DNA and slow down its dynamics. Theo- retical studies are motivated to obtain more quantitative relationships between the size of channels and resultant DNA physical properties, often expressed as scaling relationships. The dependence of DNA Fig. 2. (Top) Illustration of the steps in genome mapping. Adapted from Wang et al. [32] Fig. 1. Schematic illustration of single DNA molecules in square/rectangular channels and with permission. (Middle) examples of confined λ-DNA with sites labelled. The DNA slit-like channels. The left-bottom image shows experimental results [2] of the averaged molecules are coated with cationic-neutral diblock polypeptides to increase stretching intensity of λ-DNA in the 30 nm × 40 nm, 60 nm × 80 nm, 80 nm × 80 nm, and are confined in 150 × 250 nm2 channels. The scale bar is 5 μm. Adapted from Zhang 140 nm × 130 nm, 230 nm × 150 nm, 300 nm × 440 nm, 440 nm × 440 nm channels et al. [91] with permission. (Bottom) more examples of confined DNA with sites (left to right). The right-bottom image shows experimental results of 2λ-DNA in a labelled. Image of a single field of view 73 × 73 μm [2]. Adapted from Lam et al. [9] with 545 nm tall slit-like channel [29]. permission. 82 L. Dai et al. / Advances in Colloid and Interface Science 232 (2016) 80–100 extension on channel size has been measured in experiments [2,37–41] There is a good description of these four length parameters for con- and simulations [42–47], and compared with the predictions by Odijk fined DNA in a review paper by Reisner et al. [92],andsohereweonly theory [48] and de Gennes theory [49]. These studies have led to the de- briefly introduce these parameters. Realistic values for these four pa- velopment of theories beyond the classic Odijk and de Gennes theories, rameters are as follows. The persistence length depends on the ionic including the theory for the extended de Gennes regime [50] and the strength of the experimental buffer and other factors, while the typical backfolded Odijk regime [51–53]. The ionic strength dependence of value is 50 nm [98,99]. The definition of persistence length and how DNA extension in nanochannels has also been investigated by experi- persistence length is related to the bending stiffness and orientational ments [4,6,39]. The effects of crowding agents on the conformations of correlation can be found in standard polymer physics textbooks [100, DNA were found to be altered by nanochannels using experiments 101]. An effective chain width, w, is used to take account of the hardcore [54,55] and simulations [56,57]. The force–extension relationship and the electrostatic repulsions between DNA segments, ranging from [58–61] and fluctuation in extensions [62–65] in confinement have 10 nm to 3 nm for ionic strengths from 10 mM to 1 M [97]. The DNA been also explored. While most experiments involve linear DNA, circu- molecules used in experiments [2,4,38] are usually λ-DNA or T4-DNA, lar DNA molecules have been used in experiments [66,67] and simula- with contour lengths of approximately 16 μmand56μm, respectively. tions [68,69] as well. In addition to static properties, the dynamic These contour lengths will be increased by florescence labeling [99].In properties of confined DNA,
Details
-
File Typepdf
-
Upload Time-
-
Content LanguagesEnglish
-
Upload UserAnonymous/Not logged-in
-
File Pages21 Page
-
File Size-