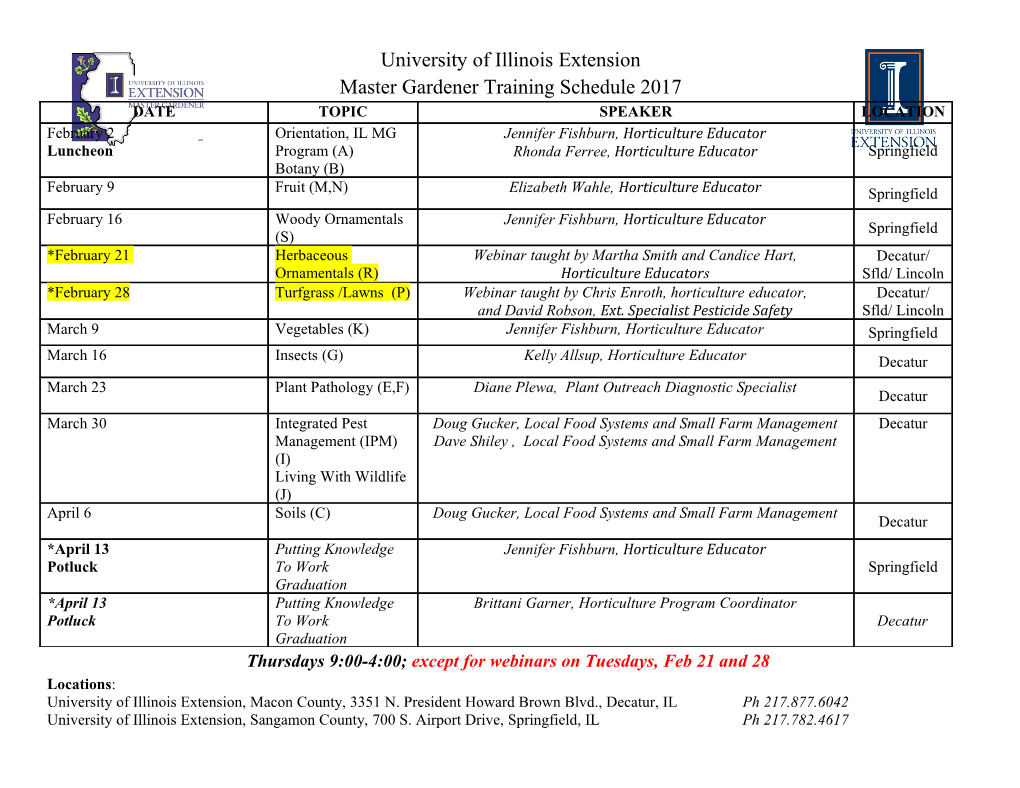
Thermodynamic Modeling and Experimental Investigation of Operating Conditions for a SOFC/GT Hybrid Power Plant Von der Fakultät Energie-, Verfahrens- und Biotechnik der Universität Stuttgart zur Erlangung der Würde eines Doktor-Ingenieurs (Dr.-Ing.) genehmigte Abhandlung Vorgelegt von Mike Steilen aus Bremen Hauptberichter: Prof. Dr. rer. nat. K. Andreas Friedrich Mitberichter: Prof. Dr. techn. Günter Scheffknecht Tag der mündlichen Prüfung: 24.05.2019 Institut für Gebäudeenergetik, Thermotechnik und Energiespeicherung der Universität Stuttgart 2019 Acknowledgments I thank Prof. Andreas Friedrich, Prof. Josef Kallo and Syed Asif Ansar for giving me the opportunity to perform research on this interesting topic. I also thank Prof. Günter Scheffknecht for co-supervising my work and Prof. André Thess for occasional scientific impulses. Special thanks go to my colleagues Moritz Henke, Christian Schnegelberger and Costanza Saletti. To Moritz for being an exceptional scientific adviser and an essential discussion partner for each and every topic conceivable. To Christian for clearing all obstacles related to equipment, lab work and bureaucracy as well as for handling suppliers and student assistants. To Costanza for being the most productive and outstanding master student imaginable, providing essential support for heat transfer modeling in this work. Another special thanks go to Miriam Steinhauer and Thomas Krummrein. To Miriam for providing insights into the mystery of electrochemical impedance spectroscopy and for the reign over spelling and commas in this dissertation, not forgetting to mention her black humor that saved me often and suits me well. To Thomas for innumerable hours of discussion and support with relation to modeling, MATLAB and the solution of numerical problems. Caroline Willich, Marc Riedel, Marc Heddrich, Srikanth Santhanam, Marius Tomberg, Lorenz Hajek and Enrico Ajanic receive my thanks for interesting discussions and support throughout our collective efforts to make the hybrid power plant real. I further thank André Schneider and Jörg Weiss-Umgethüm for support and advice on programming. I also thank my colleagues Melanie Herbst, Timo Lingstädt, Martina Hohloch and Anna Marcellan from the DLR Institute of Combustion Technology for supporting me with regard to gas turbine details. iii Abstract The SOFC/GT hybrid power plant is a promising technology to answer the chal- lenges arising from the transition of a fossil energy based and centralized power supply system to a renewable energy based and distributed power supply system. These challenges include high electrical efficiency, fuel flexibility, operational stability, security in power supply, good part-load performance and fast response to load changes. This thesis investigates operating limitations and heat transfer effects as well as stack performance and ambient conditions variations by means of a modular and computationally efficient 0D system model. The model allows for stationary and transient simulations. Model parameters are based on a real hybrid power plant that is currently under commissioning at DLR in Stuttgart. The particular component models are based on experimental data of different level of detail or factory acceptance test protocols from suppliers, where possible. If experimental results are unavailable, component parameters are based on actual design and material specifications to allow the best model parametrization and thus best prediction of operating characteristics possible. The comparison of adiabatic and non-adiabatic simulation results emphasize the importance of proper heat transfer considerations. The consecutively per- formed heat transfer variation simulations support this correlation. The effects on efficiency are significant, as expected, while the operating range is severely affected by heat transfer effects, as well. An electrical efficiency (HHV) loss of about 4 percentage points is noticed in contrast to the adiabatic results, whereas the operating range is expanded by about 2 kW in high power range due to relaxed cooling air requirements in the non-adiabatic scenario. The electrical efficiency (HHV) remains above 0.53 in the operating range of around 17 kW to 39 kW, peaking short of 0.56. The stack performance variation has only moderate influence on electrical efficiency where a gain in electrical efficiency (HHV) of up to 4 percentage points is observed with stack performance increase. However, stack performance v degradation imposes a significant system constraint, in particular for the high power operating range. The maximum power is reduced from 39 kW down to 24 kW, while the electrical efficiency (HHV) is reduced by about 2 percentage points. The ambient conditions variation refers to temperature and pressure variations, while Central European climatic conditions are assumed. The temperature variation shows a high power operating range constraint of about 5 kW once very low temperatures are investigated. The investigated pressure range shows quite similar results. However, the isothermal power range is reduced by about 60 % for the temperature variation while the impact of pressure variation results in a reduction of about 10 %. The changes in electrical efficiency (HHV) are limited in the range below 1 percentage point. The system is exposed to a transient daily ambient temperature profile, chosen from historic weather data to form a challenging scenario. However, the system does not show a significant response to the imposed daily temperature profile, indicating high operating stability for Central European climatic conditions. Eventually, the system is exposed to a challenging combined power and tem- perature profile. The isothermal power reduction by about 25 % is performed in less than 5 min while further power reduction to 50 % requires a stack tempera- ture adaption for about 1.7 h. The system follows the profile without problems, however, reaching steady state after the temperature change requires about a week’s time due to the heat stored within the system and the related surface losses. Altogether, this work allows to investigate the details of system characteristics and operating restrictions for the represented hybrid power plant. It allows to understand the effects imposed by internal and external system challenges and can predict hazardous operating regimes, to be handled with care in the real pilot power plant. vi Zusammenfassung Hybridkraftwerke aus Festoxidbrennstoffzellen und einer Gasturbine sind eine erfolgversprechende Technologie um den Herausforderungen der Energiewende zu begegnen. Die Energiewende erfordert eine Anpassung von zentralisierter hin zu dezentralisierter Infrastruktur sowie die Einbindung erneuerbarer Energieträger für die Bereitstellung elektrischer Energie. Das Hybridkraftwerk zeichnet sich durch hohe Effizienz, Flexibilität für den Einsatz unterschiedlicher Brennstoffe, Betriebssicherheit und -stabilität, die Fähigkeit Teillast-Szenarien abzudecken sowie die Fähigkeit die kurzfristigen Schwankungen durch wind- und solarener- giebasierte Energiebereitstellung auszugleichen aus. Diese Arbeit untersucht die Eigenschaften sowie die Betriebsbedingungen und -grenzen des genannten Hybridkraftwerks auf Basis eines modularen und recheneffizienten 0D-Systemmodells. Das Modell ermöglicht sowohl stationäre als auch transiente Untersuchungen. Im Speziellen werden der Transport von Wärme innerhalb des Systems und über die Systemgrenzen hinweg, sowie Änderun- gen der Leistungsdichte der Brennstoffzellen, der Einfluss von atmosphärischen Umgebungsvariablen sowie die transiente Systemantwort auf Laständerungs- szenarien untersucht. Die Parameter des Modells sind angelehnt an ein reales Hybridkraftwerk, das derzeit am DLR in Stuttgart in Betrieb genommen wird. Die enthaltenen Komponentenmodelle sind mit Hilfe von experimentellen Untersuchungen, auf Basis der Inbetriebnahmeergebnisse und teilweise durch Herstellerangaben parametriert. Im Fall von fehlenden experimentellen Ergebnis- sen werden die konkrete Beschaffenheit und die Materialspezifikationen für die Komponentenparameter herangezogen, um die voraussichtlichen Betriebseigen- schaften bestmöglich zu beschreiben. Eine Gegenüberstellung der adiabaten und nicht-adiabaten Simulationser- gebnisse, erweitert um die Variation der Wärmetransferparameter, zeigt den signifikanten Einfluss auf die elektrische Effizienz und den Betriebsbereich sowie weitere Systemdetails. Die untersuchten Wärmeverluste führen zu einem Verlust von ca. vier Prozentpunkten für den elektrischen Wirkungsgrad (HHV). Dabei vii wird der Betriebsbereich durch die Wärmeverluste um ca. zwei Kilowatt im obe- ren Leistungsbereich erhöht. Dies ist auf die Kühlwirkung durch Wärmeverluste und das damit verschobene Eintreten der Drehzahllimitierung des Gasturbinen- kompressors zurückzuführen. Die elektrische Effizienz (HHV) des Gesamtsystems im Standard-Szenario liegt oberhalb von 0,53 für den gesamten Betriebsbereich von 17 kW bis 39 kW und erreicht bei knapp 0,56 seinen Spitzenwert. Die potentielle Leistungssteigerung des Brennstoffzellenmoduls um ca. 30 % führt zu einem zwei bis vier Prozentpunkte höheren elektrischen Wirkungsgrad bei ähnlichem Betriebsbereich. Die Leistungsdegradation um ca. 30 % führt zu einer signifikanten Verringerung der Leistung von 39 kW auf 24 kW und dabei zu einem um ca. zwei Prozentpunkte verminderten elektr. Wirkungsgrad (HHV). Die Variationen der Umgebungsbedingungen beziehen sich auf Temperatur- und Druckschwankungen auf der Grundlage des mitteleuropäischen Klimas. Die Temperaturvariation zeigt
Details
-
File Typepdf
-
Upload Time-
-
Content LanguagesEnglish
-
Upload UserAnonymous/Not logged-in
-
File Pages151 Page
-
File Size-