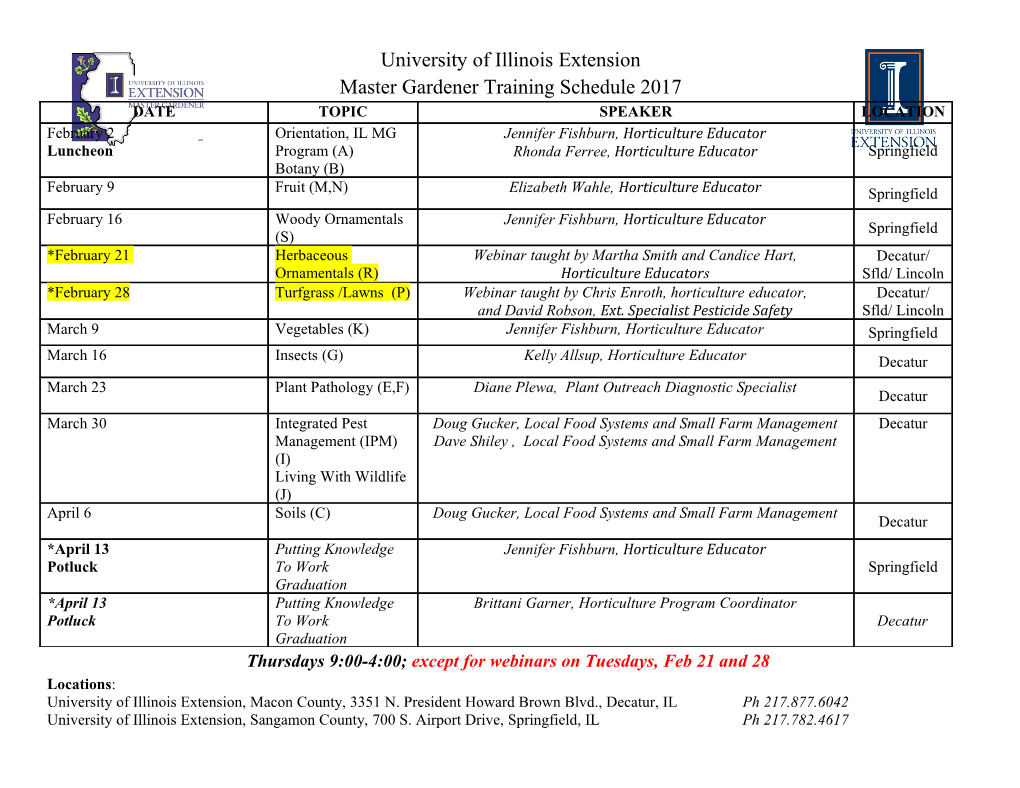
A counterexample to Las Vergnas’ strong map conjecture on realizable oriented matroids Pei Wu email: [email protected] April 23, 2019 Abstract The Las Vergnas’ strong map conjecture, asserts that any strong map of oriented matroids f : M1 → M2 can be factored into extensions and contractions. This conjecture is known to be false due to a construction by Richter-Gebert, he finds a strong map which is not factorizable, however in his example M1 is not realizable. The problem that whether there exists a non-factorizable strong map between realizable oriented matroids still remains open. In this paper we provide a counterexample to the strong map conjecture on realizable oriented matroids, which is a strong map f : M1 →M2, M1 is an alternating oriented matroid of rank 4 and f has corank 2. We prove it is not factorizable by showing that there is ′ ′ no uniform oriented matroid M of rank 3 such that M1 →M →M2. 1 Background The strong map conjecture, firstly posed by Las Vergnas[1], asserts that any strong map of oriented matroids f : M1 →M2 can be factored into extensions and contractions. It is known that the conjecture holds for ordinary matroids [6]. And for oriented matroids a counterexample has been constructed by Richter- Gebert [11]. However, M1 is not realizable in Richter-Gebert’s construction. The problem that whether Las Vergnas’ conjecture holds when M1 is realizable still remains open. In this paper, we will present an counterexample disproving this conjecture. Theorem 1. There is a strong map f : M1 → M2 with M1 being an real- izable oriented matroid of rank 4 on 8 elements and f corank 2, which is not arXiv:1803.06825v2 [math.CO] 22 Apr 2019 factorizable into extensions and contractions. Las Vergnas’ conjecture on realizable oriented matroids has its own sig- nificance as a part of the ”combinatorial Grassmannian” program [10]. The program is stimulated by pioneering works of Gelfand and MacPherson [5, 8], in [5] they proposed a formula that calculates rational Pontrjagin classes of a differentiable manifold from combinatorial data. In their proof they make use of a modified formulation of Chern-Weil theory. So it is not possible to calculate any Z/pZ-characteristic classes following same argument. A possible way to remedy this deficit is to adopt the definition of characteristic classes via Grass- mannians. Let’s recall some standard facts of characteristic classes (see [3] or 1 [9] for a comprehensive treatment). Let p : E → B be a real vector bundle on a manifold B, a characteristic class of the bundle is an invariant taking value in cohomology ring H∗(B) of certain coefficients. If p is a Rk-bundle, there is ∞ a canonical map (up to isotopy) from B to the infinite real Grassmannian Gk , coined Gauss map, and characteristic classes are pull-backs of certain cohomol- ogy classes on infinite real Grassmannian. Such definition is purely topological, so one would expect that we are able to rewrite this definition using combina- torial data with less effort. MacPherson[10] suggests the following object as a n substitute of Gk(R ): the (chain complex of) poset of all oriented matroids of rank k on n elements, ordering with respect to weak maps, called MacPherso- nian and denoted as MacP(n, k). Let F n be the free oriented matroid of rank n, MacP(n, k) is the poset of rank k strong image of F n. One can obtain more general object by substituting F n with an arbitrary rank n oriented matroid M (one can assume M is realizable for our purpose), called OM-Grassmannian and denoted as Gk(M). Combinatorial Grassmannian program is the study of n homopoty type of Gk(M). The conjecture that Gk(M) and Gk(R ) are homo- topy equivalent has been disapproved by Gaku Liu [7]. And whether MacP(n, k) n and Gk(R ) are homotopy equivalent still remains open. The Las Vergnas’ strong map conjecture is related with combinatorial Grass- mannian program in the following way: the (non-compact) Stiefel manifold n Vk(R ) is the set of all k-tuples of linearly independent vectors, there is a sur- n n jective mapping p : Vk(R ) → Gk(R ) by sending the k-tuples to the linear n −1 space they span. For every pt ∈ Gk(R ), p ({pt}) is isomorphic to GL(k, R), n n so Vk(R ) can be viewed as a principal GL(k, R)-bundle over Gk(R ). The ori- ented matroid counterpart of Stiefel manifold is defined as follows: let M be an oriented matroid of rank n, the OM-Stiefel space Vk(M) is defined as all ”non- degenerate” n−k extensions, i.e. if the set of new elements is S, the contraction M/S should has rank k. So there is a poset mappingp ˜ : Vk(M) → Gk(M) de- fined by contracting S. A natural problem is, is preimage of every point is homotopic to GL(k, R)? Note that the Las Vergnas’ strong map conjecture would implies the subjectivity ofp ˜. Our counterexample indicates that there is a point with empty preimage. 2 Oriented Matroids For completeness we will include a brief introduction to the theory of oriented matroids, in which we try to cover most conventions and facts we use in this paper, one could refer to [2] for a detailed treatment. Datum of oriented matroid can be encoded by circuits, vectors, cocircuits, covectors, topes or chirotope. Let E be the ground set. Circuits, vectors, co- circuits, covectors, topes are all signed vectors on E. A signed vector X is a mapping X : E → {−1, 0, 1}. X−1(1) and X−1(−1) are denoted as X+ and X−, respectively. We will use two ways to write the signed vectors, for example when E = {1,..., 5}, X+ = {1, 3} and X− = {2, 4}, X = (+ − + − 0) or X =123¯ 4.¯ 0 is the signed vector X with X+ = X− = ∅, 1 is the signed vector X with X+ = X. If X is a signed vector, define −X to be the signed vector with (−X)(i)= −X(i), which is called the opposite of X. Given a set of signed vectors X , reorientation of an element e ∈ E is the operation reversing values of X(e) for all signed vectors X ∈ X . The support of a signed vector is defined as 2 X+ ∪ X−, denoted as X, the size of X is defined as the size of support, signed vector X has full support iff X = E. Two signed vectors Y and Z are perpen- dicular iff in their component-wise products X, X+ and X− are all empty or all non-empty, written as Y ⊥ Z. There is a natural partial ordering on signed vectors: X X′ iff X+ ⊆ X′+ and X− ⊆ X′−. If E′ ⊆ E, restriction of X on ′ ′ ′ E is a signed vector on E , defined as X|E′ (i)= X(i) for i ∈ E . The chirotope is an anti-symmetric mapping χ : Er → {1, 0, −1}, in which r = r(M) is the rank of the oriented matroid. An oriented matroid can be encoded by a set of circuits, or cocircuits, etc, satisfying certain sets of axioms ([2] Chapter. 3). For completeness, we include the covector axiomatization of oriented matroids here: Definition 1. An oriented matroid is a pair M = (E, L), covectors L is a set of signed vector on E such that: 1. 0 ∈ L 2. X ∈ L =⇒ −X ∈ L 3. X, Y ∈ L =⇒ X ◦ Y ∈ L 4. (covector elimination) X, Y ∈ L, e ∈ S(X, Y ) =⇒ there exist Z ∈ L such that Z(e)=0 and Z(f) = (X ◦ Y )(f) for f 6∈ S(X, Y ). In which S(X, Y ) := {e ∈ E|X(e) = −Y (e) 6= 0} and X ◦ Y is the signed vector defined as X(e), if X(e) 6=0 (X ◦ Y )(e)= (Y (e), otherwise r− A finite set of points E = {v1,..., vn} in affine space R 1 is a point con- figuration if their affine closure is Rr−1, we can associate it with an oriented matroid M. Each affine dependency λivi = 0, λi = 0 defines a vector X + − of M by X = {vi|λi > 0} and X = {vi|λi < 0}. Geometrically this implies the convex hull of X+ and X− are intersectingP atP interior points. And each r∗ w ∈ R , a ∈ R defines a covector X of M such that X+ = {vi| hvi, wi > a}, − − X = {vi| hvi, wi < a}. That is, X+ and X lie in two half-spaces cut by hyperplane {vi| hvi, wi = a}. Circuits are non-zero -minimal vectors and co- circuits are non-zero -minimal covectors and topes are -maximal covectors. The chirotope is an alternating function on Er, χ : Er → {−1, 0, 1}, defined by χ(i1,...,ir) = sign(det(vi1 − vir ,..., vir−1 − vir )) (−χ is considered to be same chirotope as χ ). An oriented matroid is realizable iff it arises in this way for some {ve : e ∈ E}, up to reorientation of elements. One could verify that every vector is perpendicular to every covector, which is a property also holds for non-realizable oriented matroids. An oriented matroid is acyclic iff 1 is a covector. An oriented matroids is uniform iff χ(i1,...,ir) 6= 0 for all i1,...,ir distinct. In an uniform oriented matroid the size of circuits are always r + 1 and size of cocircuits are always r n n−r. Define Φr(n) := i=0 i , the number of topes is 2Φr−1(n−1). Actually the converse is also true by [4], which provides an alternative axiomatization of uniform oriented matroid,P for which will be useful for enumerating oriented matroids.
Details
-
File Typepdf
-
Upload Time-
-
Content LanguagesEnglish
-
Upload UserAnonymous/Not logged-in
-
File Pages7 Page
-
File Size-