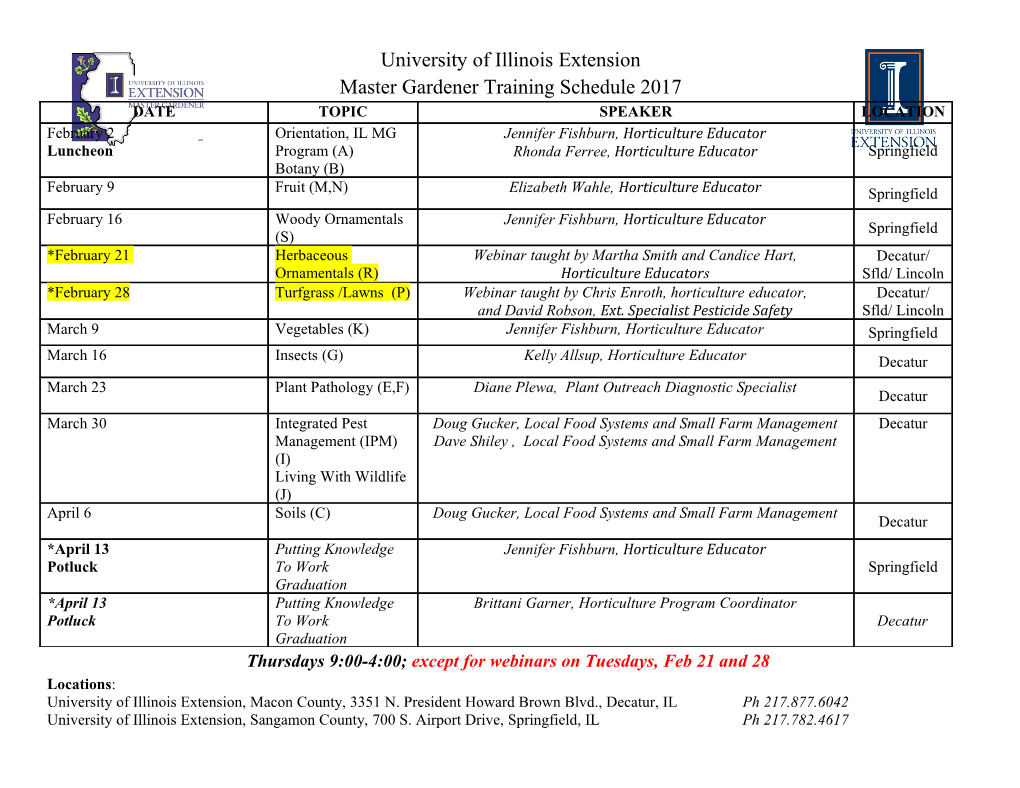
Red and Fallow Deer Determine the Density of Ixodes Ricinus Nymphs Containing Anaplasma Phagocytophilum Katsuhisa Takumi ( [email protected] ) Centre for Zoonoses and Environmental Microbiology Centre for Infectious Disease Control National Institute for Public Health and the Environment (RIVM) Bilthoven The Netherlands Tim Hofmeester Swedish University of Agricultural Sciences Faculty of Natural Resources and Agricultural Sciences Hein Sprong Centre for Zoonoses and Environmental Microbiology Centre for Infectious Disease Control National Institute for Public Health and the Environment (RIVM) Bilthoven The Netherlands Research Keywords: Ixodes ricinus nymphs, Anaplasma phagocytophilum, phagocytophilum, anaplasmosis Posted Date: October 26th, 2020 DOI: https://doi.org/10.21203/rs.3.rs-96286/v1 License: This work is licensed under a Creative Commons Attribution 4.0 International License. Read Full License Version of Record: A version of this preprint was published on January 19th, 2021. See the published version at https://doi.org/10.1186/s13071-020-04567-4. 1 Red and fallow deer determine the density of Ixodes ricinus 2 nymphs containing Anaplasma phagocytophilum 1, 2 1 3 Katsuhisa Takumi ✉, Tim R. Hofmeester , and Hein Sprong 4 1 Centre for Zoonoses and Environmental Microbiology Centre for Infectious Disease Control 5 National Institute for Public Health and the Environment (RIVM) Bilthoven The Netherlands 6 2 Department of Wildlife Fish and Environmental Studies Swedish University of Agricultural 7 Sciences Skogsmarksgränd 7 907 36 Umeå Sweden 8 ✉ Correspondence: Katsuhisa Takumi <[email protected]> 9 1 10 Abstract 11 Background: The density of Ixodes ricinus nymphs infected with Anaplasma phagocytophilum 12 is one of the parameters that determines the risk for humans and domesticated animals to 13 contract anaplasmosis. For this, I. ricinus larvae need to take a blood meal from free-ranging 14 ungulates, which are competent hosts for A. phagocytophilum. 15 Methods: Here, we compared the contribution of four free-ranging ungulate species, red deer 16 (Cervus elaphus), fallow deer (Dama dama), roe deer (Capreolus capreolus), and wild boar (Sus 17 scrofa) to A. phagocytophilum infections in nymphs. We used a combination of camera and live 18 trapping to quantify the relative availability of vertebrate hosts to questing ticks in nineteen 19 Dutch forest sites. Additionally, we collected questing I. ricinus nymphs and tested these for the 20 presence of A. phagocytophilum. Furthermore, we explored two potential mechanisms that could 21 explain differences between species: 1) differences in larval burden, which we based on data 22 from published studies, and 2) differences in associations with other, non-competent hosts. 23 Results: Principal component analysis indicated that the density of A. phagocytophilum infected 24 nymphs (DIN) was higher in forest sites with high availability of red and fallow deer, and to a 25 lesser degree roe deer. Initial results suggest that these differences are not a result of differences 26 in larval burden, but rather differences in associations with other species or other ecological 27 factors. 28 Conclusions: These results indicate that the risk for contracting anaplasmosis in the Netherlands 29 is likely highest in the few areas where red and fallow deer are present. Future studies are needed 30 to explore the mechanisms behind this association. 2 31 Background 32 Anaplasma phagocytophilum is the causative agent of human granulocytic anaplasmosis (HGA), 33 and it also causes disease and economic losses in domesticated animals [1–3]. The first human 34 case in Europe was reported in 1995. Since, HGA cases have only been occasionally reported 35 throughout Europe [4]. It is unclear to what extent HGA poses a health burden in Europe: 36 epidemiological data on the disease incidence and disease burden is either incomplete or lacking 37 from most European countries [2]. The non-specificity of the reported symptoms, poor 38 diagnostic tools and lack of awareness of public health professionals may also complicate these 39 estimations [5]. Anaplasma phagocytophilum is transmitted by the bite of an infected tick [6,7]. 40 The main vector in Europe is Ixodes ricinus, which also transmits Borrelia burgdorferi sensu 41 lato, the causative agent of Lyme borreliosis, and several other pathogens [8]. The geographic 42 spread and density of I. ricinus infected with A. phagocytophilum is an important determinant of 43 the disease risk [9,10]. Anaplasma phagocytophilum seems to appear in all countries across 44 Europe with infection prevalence in nymphs (NIP) varying between and within countries from 0 45 to 25% [1]. Understanding which factors determine the spatial and temporal distribution of I. 46 ricinus infected with A. phagocytophilum is needed for risk assessments and for formulating 47 possible intervention strategies. 48 Variations in the density of questing I. ricinus infected with A. phagocytophilum (DIN) have 49 partly been attributed to environmental factors such as differences in weather conditions [11,12], 50 habitat characteristics [13], as well as vertebrate communities [14]. Whereas small mammals and 51 birds are considered to feed the majority of immature I. ricinus, ungulates act as their main 52 propagation host [15]. It is however, still unclear which host species form the main reservoir for 53 A. phagocytophilum, and therefore contribute most to the density of infected ticks. Anaplasma 3 54 phagocytophilum has been found to infect many vertebrate species [1], but its genetic diversity 55 indicates that there are multiple genetic variants, or ecotypes, with distinct, but overlapping 56 transmission cycles, pathogenicity or geographical origin [16–18]. A variety of wildlife species, 57 like red deer (Cervus elaphus), fallow deer (Dama dama) wild boar (Sus scrofa), and European 58 hedgehog (Erinaceus europaeus), are harbouring A. phagocytophilum variants that can cause 59 disease in humans and domesticated animals, whereas roe deer (Capreolus capreolus), rodents 60 and birds seem to carry genetic variants that have until now not been associated with human 61 disease [17]. 62 About two-thirds of tick bites reported in the Netherlands are I. ricinus nymphs [19]. Therefore, 63 the density of questing nymphs infected with A. phagocytophilum (DIN) is an important 64 ecological parameter that, together with the level of human exposure, determines tick-borne 65 disease risk [9,20]. The DIN is calculated by multiplying the density of questing I. ricinus 66 nymphs (DON) by nymphal infection prevalence (NIP). The transmission of A. phagocytophilum 67 predominantly relies on horizontal transmission between ticks and vertebrate hosts and on 68 transstadial transmission in its vectors, as vertical (transovarial) transmission has not been 69 documented for I. ricinus. Therefore, an I. ricinus larvae needs to take a blood meal from an 70 infected vertebrate host to become an infected I. ricinus nymph. The availability of (infected) 71 vertebrates to questing larvae generally drives the density of (infected) I. ricinus nymphs 72 [14,21,22]. 73 Using data from a cross-sectional study estimating the availability of hosts with camera and live 74 traps in nineteen Dutch forest sites, we quantified a moment when a questing tick encounters an 75 ungulate, the probability of this event predicts both I. ricinus nymphal density, and A. 76 phagocytophilum DIN [14]. However, the reported association for A. phagocytophilum DIN left 4 77 a relatively large proportion of the variation among sites unexplained, which could be due to the 78 grouping of four ungulate species. The group of ungulates considered consisted of four species 79 that differed in their ecology and potentially in their ability as hosts for I. ricinus and A. 80 phagocytophilum. 81 Here, we present a re-analysis of the A. phagocytophilum data from the cross-sectional study to 82 disentangle the role of the four ungulate species in determining A. phagocytophilum DIN. We 83 first applied a principal component analysis to the camera and live trapping data to test if 84 availability of any of the four ungulate species or combinations of species was associated with A. 85 phagocytophilum DIN. Second, we used simple mathematical models to explore two potential 86 mechanisms that could explain differences between species: 1) differences in I. ricinus larval 87 burden, and 2) potential associations of the different ungulate species with alternative 88 incompetent host species. 89 Methods 90 Cross-sectional study 91 We made use of data from an extensive field survey that was carried out in nineteen 1-ha sites 92 located in forested areas in the Netherlands in 2013 and 2014. Data were collected on the density 93 of questing I. ricinus (blanket dragging), vertebrate communities (camera and live trapping), and 94 on the infection rates of tick-borne pathogens (qPCR detection). The sites, methodologies, and 95 the data have been described elsewhere as well as a series of detailed analyses [14,22–24]. 5 96 Host attribution 97 We arranged the encounter probabilities for all forest sites (n=19) and vertebrate species (n=32) 98 into a matrix having 19 rows and 32 columns. We further arranged A. 19×32 99 phagocytophilum퐀 ∈ DIN ℝ into a vector matching the order of the forest sites along the rows of . 100 To attribute A. phagocytophilum DIN푏 to an assemblage of 32 vertebrate species, we factored the퐀 101 matrix into two orthogonal matrices 102 퐀 19×19 32×32 1 2 19 1 2 32 103 and a diagonal퐔 matrix = [푢 , 푢 , … , 푢 ] ∈with ℝ the singular and 퐕 values = [푣 , 푣 , … , 푣 ] ∈ ℝ and the 19×32 104 remaining entries equal횺 to ∈ zero. ℝ Theorem 2.5.2 [25] proves that휎1 ≥ 휎2 … ≥ 휎19. The≥ 0 column vectors 푇 105 and are principal components, also known as singular vectors.퐀 = 퐔횺퐕 106 The푢푖 A. 푣phagocytophilum푖 DIN increased at each forest site due to the first principal components 107 by the amount (Theorem 5.5.1 [25]) 108 푢1 ⋅ 푏 19 퐀푣1 ∈ ℝ . (1) 109 We attributed Eq.(1) to roe deer because휎1 the highest contribution from the first principal 110 component comes from roe deer.
Details
-
File Typepdf
-
Upload Time-
-
Content LanguagesEnglish
-
Upload UserAnonymous/Not logged-in
-
File Pages36 Page
-
File Size-