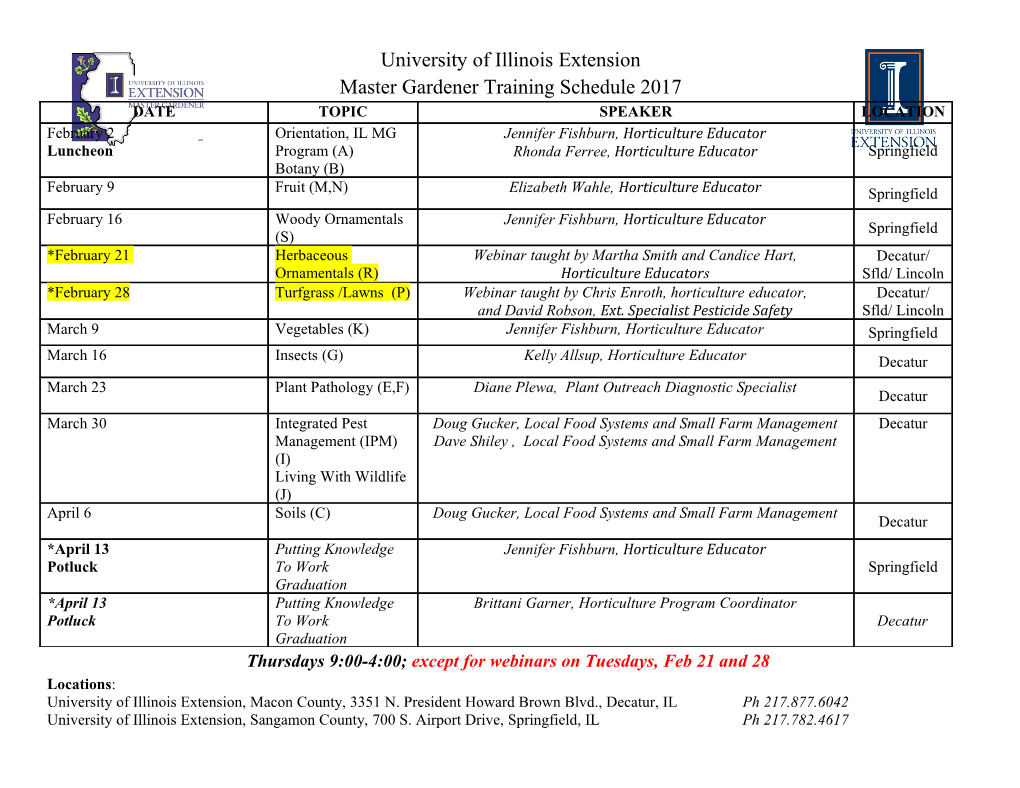
Vol 463 | 4 February 2010 | doi:10.1038/nature08775 LETTERS A ground-based near-infrared emission spectrum of the exoplanet HD 189733b Mark R. Swain1, Pieter Deroo1, Caitlin A. Griffith2, Giovanna Tinetti3, Azam Thatte5, Gautam Vasisht1, Pin Chen1, Jeroen Bouwman6, Ian J. Crossfield4, Daniel Angerhausen7, Cristina Afonso6 & Thomas Henning6 Detection of molecules using infrared spectroscopy probes the con- curve, beginning approximately one hour before the onset of ingress ditions and compositions of exoplanet atmospheres. Water (H2O), and ending approximately one hour after the termination of egress. methane (CH4), carbon dioxide (CO2), and carbon monoxide (CO) HD 189733 was observed at two positions (A and B) on the spectro- have been detected1–5 in two hot Jupiters. These previous results graph entrance slit using an AB BA sequence. Spectral calibration was relied on space-based telescopes that do not provide spectroscopic done using the internal instrument calibration arc lamps. To minimize capability in the 2.4–5.2 mm spectral region. Here we report the effects of seeing-based modulation of the measured flux density, a ground-based observations of the dayside emission spectrum for 1.6-arcsecond slit width was used. The SpeX instrument was con- HD 189733b between 2.0–2.4 mm and 3.1–4.1 mm, where we find a figured to observe between 1.9 and 4.2 mm at an average spectral reso- bright emission feature. Where overlap with space-based instru- lution of 470. The slit length for these observations was 15 arcseconds, ments exists, our results are in excellent agreement with previous and the sky background and source were measured simultaneously. measurements2,6. A feature at 3.25 mm is unexpected and difficult We developed a novel calibration method (described in the to explain with models that assume local thermodynamic equi- Supplementary Information) for systematic error removal and sub- librium (LTE) conditions at the 1 bar to 1 3 1026 bar pressures sequent detection of the eclipse. This method is based on an iterative typically sampled by infrared measurements. The most likely approach to removing systematic errors while the secondary eclipse is explanation for this feature is that it arises from non-LTE emission extracted by computing the self-coherent spectrum of groups of 13 from CH4, similar to what is seen in the atmospheres of planets in channels . Although we have not reached the theoretical noise level our own Solar System7–9. These results suggest that non-LTE effects and further improvements are possible, this approach is highly suc- may need to be considered when interpreting measurements of cessful in detecting the secondary eclipse and, significantly, does not strongly irradiated exoplanets. require the use of priors (for example, system ephemeris). We vali- Spectroscopic detection of molecules in exoplanet atmospheres is dated the calibration/spectral extraction method by comparing the a relatively recent development. High-precision spectroscopy data in ground-based results to previous space-based measurements of combination with spectral retrieval techniques enables the deter- HD 189733b. Between 2.0 and 2.4 mm, the ground-based results mination of the atmospheric temperature profile and composition; clearly show the CO2 absorption feature present in the exoplanet this, in turn, provides some degree of knowledge about the atmo- atmosphere and agree well with previous Hubble measurements2 spheric chemistry via molecular abundance ratios. Combinations of (see Fig. 1). In the 3.1–4.1 mm region, we compared the ground-based 6 the molecules H2O, CH4,CO2, and CO have been detected in the hot- results with Spitzer photometry measurements , by integrating the Jupiter-type exoplanets HD 189733b and HD 209458b during prim- ground-based spectrum over the Spitzer 3.6-mm filter pass band, and ary eclipse1,2 and/or secondary eclipse3–5 events (when the planet we find agreement at the 1s level. Between 3.1 and 4.1 mm, the passes either in front of or behind the stellar primary). In the case ground-based observations provide a unique capability, and it is here of HD 189733b, the primary eclipse spectrum probes the terminator that we find emission features far exceeding those characteristic of region and the secondary eclipse spectrum probes the dayside region other wavelengths in the HD 189733b dayside spectrum (see Fig. 2). of the planet’s atmosphere, thus permitting atmospheric conditions We investigated the plausibility of purely thermal emission by in these two regions to be compared. Temporal variability has also calculating the brightness-temperature spectrum; these values indi- been detected in the mid-infrared emission spectrum3 and may indi- cate the atmospheric temperatures probed by the light emitted at cate that dynamical processes have a significant role in establishing each wavelength when LTE conditions (collision-dominated) apply. the atmospheric properties10,11. Until now, the detection of molecules The 3.25-mm flux density corresponds to a brightness temperature of in exoplanet atmospheres has required the stability of the Hubble and 2,700 K, which markedly exceeds the typical brightness temperatures Spitzer space telescopes. Observing exoplanet emission spectra from in HD 189733’s spectrum (see Fig. 2 inset). Prior studies3,6,11,14 and the ground is complicated by both the intrinsic variability of the our own modelling successfully explained most of the infrared spec- Earth’s atmosphere and instrument instability caused, for example, trum as thermal emission modulated by molecular opacity; these by changes in the instrument orientation as the telescope tracks. studies imply a temperature profile that decreases from roughly The spectrum we present here is based on data obtained on 11 1,600 K at 1 bar to roughly 800 K in the ,1023–1025 bar range. August 2007, using data from the SpeX instrument12 on the NASA However, these LTE models do not explain the flux density of Infrared Telescope Facility (IRTF). The observations of the 3.1–4.1 mm; modification of these models to include a tropopause HD 189733 system were timed to observe the secondary eclipse light and an appropriate absorber could explain the weak emission around 1Jet Propulsion Laboratory, California Institute of Technology, 4800 Oak Grove Drive, Pasadena, California 91109-8099, USA. 2University of Arizona, Lunar and Planetary Laboratory, Space Science Building Room 525, 1629 East University Boulevard, Tucson, Arizona 85721, USA. 3Department of Physics and Astronomy, University College London, Gower Street, WC1E 6BT, London, UK. 4Astronomy Department UCLA, 475 Portola Plaza, Los Angeles, California 90034, USA. 5Woodruff School of Mechanical Engineering, MRDC Building, Room 4111, Georgia Institute of Technology, Atlanta, Georgia 30332-0405, USA. 6Max-Planck Institute for Astronomy, Koenigstuhl 17, D-69117, Heidelberg, Germany. 7German SOFIA Institute, Institute for Space Systems, Pfaffenwaldring 3170569, Stuttgart, Germany. 637 ©2010 Macmillan Publishers Limited. All rights reserved LETTERS NATURE | Vol 463 | 4 February 2010 a 3,000 IRTF 2.07 µm 0.010 HST/NICMOS 2,500 Hubble Space Spitzer/IRAC Telescope Spitzer/IRS 2,000 IRTF IRTF data averaged 1.002 0.008 for IRAC1 pass band 1,500 0.10 2.13 µm Model spectrum ] 1,000 star F Brightness temperature (K) Brightness temperature / 500 2.19 µm 0.006 (%)] 2345678910 planet star F Wavelength (µm) F 1.000 / 0.05 planet F [ 2.26 µm 0.004 Flux ratio, [ 0.998 0.00 2.33 µm 0.002 1.8 1.9 2.0 2.1 2.2 2.3 Wavelength (µm) 0.000 0.996 b 2 3 4 5 6 7 8 9 10 11 12 13 Wavelength (µm) Spitzer/IRAC 3.04 µm IRTF 1.0 Figure 2 | Unexpectedly strong 3.25-mm emission present in the dayside 1.02 IRTF data spectrum. The brightness temperature of the 3.25-mm emission feature averaged for IRAC1 indicates the likely presence of a non-LTE emission mechanism. The dayside Time series (normalized and shifted) bandpass 0.8 emission spectrum is based on the new measurements reported in this paper (black), together with previous results from Hubble spectroscopy (red), 1.01 Spitzer spectroscopy (green), and Spitzer photometry (blue); all data are 3.34 µm (%)] 0.6 shown 61s errors. A radiative transfer model (grey) assuming LTE star F / conditions and consistent with the measurements made with the Spitzer and planet F [ Hubble space telescopes fails to describe the emission structure at 1.00 0.4 3.1–4.1 mm, and we find no plausible combination of atmospheric parameters that provides a good model of the observations under LTE conditions. The inset plot displays the brightness temperature at each 0.2 0.99 3.78 µm wavelength and shows the large temperature change needed to produce the 3.25-mm emission if LTE conditions hold. IRS, the Infrared Spectrograph; NICMOS, the Near Infrared Camera and Multi-Object Spectrometer. The 0.0 3.0 3.2 3.4 3.6 3.8 4.0 4.03 µm dotted lines are a visual aid to help draw the eye to the new IRTF data. 0.98 Wavelength (µm) 0.44 0.46 0.48 0.50 0.52 models, and (3) the prevalence of fluorescence in our own Solar Orbital phase (normalized) System, all indicate that non-LTE CH4 emission is probably present Figure 1 | Dayside spectra and secondary eclipse light curves. The ground- in the atmosphere of HD 189733b. A puzzling aspect of these data is based spectra are in excellent agreement with space-based measurements. the absence of strong emission from the CH4 n3 band P branch; a a, Comparison of the IRTF measurements (red) with previous similar effect has been detected in the upper atmosphere of Titan b measurements using the Hubble Space Telescope (green). , Comparison of (see Supplementary Information) and remains unexplained. the IRTF spectrum (red) and IRTF data averaged to the Spitzer 3.6-mm pass band (blue solid square) with the Spitzer Space Telescope 3.6-mm The 3.1–4.1-mm spectrum shows that the strong emission from the photometry measurement (blue open square).
Details
-
File Typepdf
-
Upload Time-
-
Content LanguagesEnglish
-
Upload UserAnonymous/Not logged-in
-
File Pages3 Page
-
File Size-