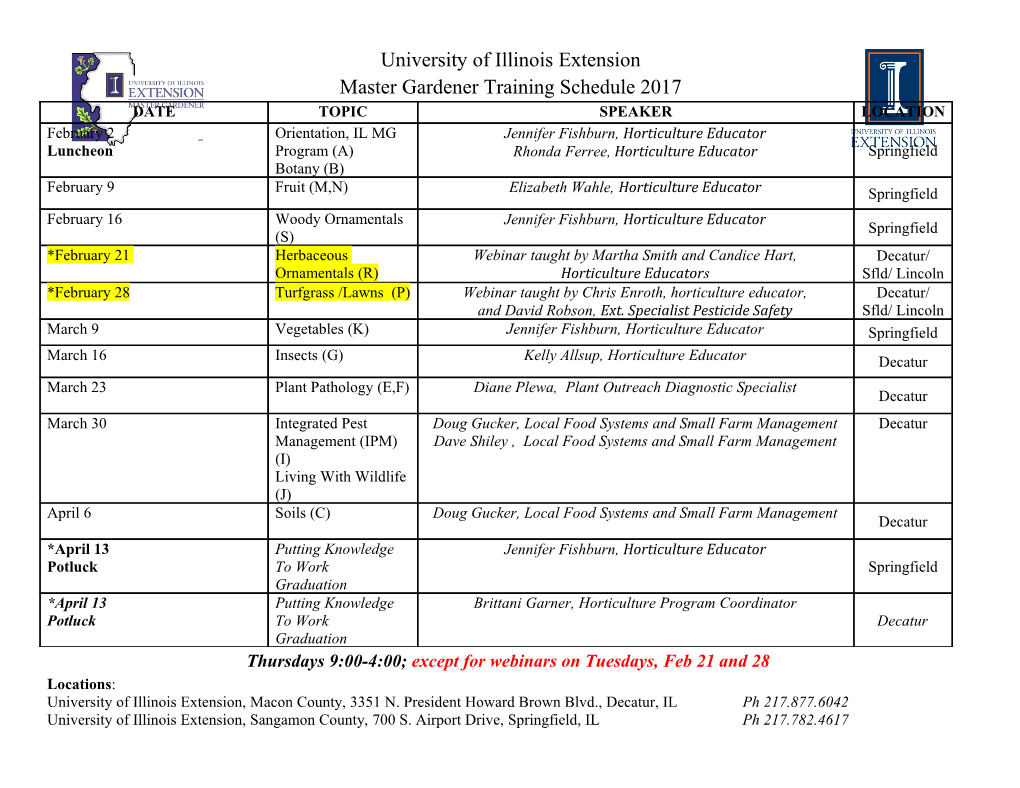
HYDROLOGICAL PROCESSES Hydrol. Process. 15, 2175–2194 (2001) DOI: 10.1002/hyp.275 Development of WEP model and its application to an urban watershed Yangwen Jia,1* Guangheng Ni,1 Yoshihisa Kawahara2 and Tadashi Suetsugi3 1 Urban River Division, Public Works Research Institute (PWRI), Asahi, Tsukuba City 305-0804, Ibaraki Prefecture, Japan 2 Department of Safety Systems Construction Engineering, Kagawa University, Kagawa 761-0396, Japan 3 River Hydraulics Division, Public Works Research Institute, Tsukuba, Japan Abstract: A distributed hydrological model, water and energy transfer processes (WEP) model, is developed to simulate spatially variable water and energy processes in watersheds with complex land covers. In the model, state variables include depression storage on land surfaces and canopies, soil moisture content, land surface temperature, groundwater tables and water stages in rivers, etc. The subgrid heterogeneity of land use is also taken into consideration by using the mosaic method. For hydrological processes, evapotranspiration is computed by the Penman–Monteith equation, infiltration excess during heavy rains is simulated by a generalized Green–Ampt model, whereas saturation excess during the remaining periods is obtained by doing balance analysis in unsaturated soil layers. A two-dimensional simulation of multilayered aquifers is performed for groundwater flow. Flow routing is conducted by using the kinematic wave method in a one-dimensional scheme. For energy processes, short-wave radiation is based on observation or deduced from sunshine duration, long-wave radiation is calculated according to temperatures, latent and sensible fluxes are computed by the aerodynamic method and surface temperature is solved by the force–restore method. In addition, anthropogenic components, e.g. water supply, groundwater lift, sewerage drainage and energy consumption, etc. are also taken into account. The model is applied to the Ebi River watershed (27 km2) with a grid size of 50 m and a time step of 1 h. The model is verified through comparisons of simulated river discharges, groundwater levels and land surface temperatures with the observed values. A comparison between water balance at present (1993) and that in the future (2035) is also conducted. It is found that the hydrological cycle in the future can be improved through the implementation of infiltration trenches for the storm water from urban canopies. Copyright 2001 John Wiley & Sons, Ltd. KEY WORDS hydrological processes; energy processes; urbanization; distributed model INTRODUCTION With the change of land surface and living styles in urban river watersheds, the hydrological cycle has been greatly changed and this change still continues or even accelerates in some watersheds. In urban rivers, discharges are increased and concentration times become shorter during flood periods. On the other hand, discharges become much less and water quality deteriorates during dry seasons. In addition, the microclimate in urban areas is also changed, e.g. the urban heat island and dry weather phenomena become more and more evident. Ensuring sustainable developments in urban areas requires understanding the real situation of water and energy budgets in watersheds and taking systematic and effective countermeasures. Distributed physically-based hydrological models can take account of spatial variations of all variables and parameters involved in the basic mathematical equations of the water flows for a watershed. In addition, the used parameters are physically measurable. Therefore, they give a detailed and potentially more correct description of the hydrological processes in the watershed than empirical and conceptual hydrological models. * Correspondence to: Y. Jia, Hydrologic Engineering, Public Works Research Institute (PWRI), Minamihara 1–6, Tsukuba City 305-8516, Ibaraki Prefecture, Japan. E-mail: [email protected] Received 3 April 2000 Copyright 2001 John Wiley & Sons, Ltd. Accepted 18 August 2000 2176 Y. JIA ET AL. With more available data, especially with the development of GIS and remote sensing technology, the study and application of these types of models will surely be promoted. Today, several popular models are of this type, such as SHE (Abbott et al., 1986), IHDM (Beven et al., 1987) and MIKE SHE (Refsgaard and Storm, 1995), etc. In this paper, the water and energy transfer processes (WEP) model developed at PWRI is described, which adds more detailed energy balance analysis in hydrological modelling. The model is based on Jia and Tamai (1998) and is improved by adding the simulation of multilayered aquifers, direct computation of groundwater outflow to rivers and simulation of infiltration trenches. The paper consists of two main parts: (1) model development and (2) its application to the Ebi River watershed. In the first part, a distributed and physically-based model is developed to explain spatially variable water and energy processes with complex land covers, which is not only applicable to natural watersheds but also applicable to urbanized watersheds. Evapotranspiration and latent heat flux are computed by combining the Penman–Monteith equation (Monteith, 1973) with the force–restore method (Hu and Islam, 1995) instead of the potential value method. More efficient and reasonable modelling of infiltration is also conducted by using a generalized Green–Ampt model (Jia and Tamai, 1997). In addition, the mosaic method (Avissar and Pielke, 1989) is used to consider the subgrid heterogeneity of land use. In the second part, the model is applied to simulate the hydrological cycle in the Ebi River watershed with a grid size of 50 m and a time step of 1 h. A 6-year simulation from 1992 to 1997 is performed. The model is verified through comparisons of simulated river discharges, groundwater levels and land surface temperatures with the observed values. The comparison of water balance at present (1993) with that in the future (2035) is also conducted and it shows the impact of urbanization. Finally, the effect of installing infiltration trenches is assessed. Although the WEP model simulates most of the hydrological processes by similar methods to the distributed physically-based models mentioned above, its main differences from those models are as follows. (1) The energy transfer processes are also simulated in detail in addition to hydrological processes. Because of its detailed consideration of heat flux partitions on land surface, the model not only enhances the computations of interception and evapotranspiration, but also is easy to couple to atmospheric models. (2) The subgrid heterogeneity of land use is considered by using the mosaic method, which is believed to be more reasonable than the usual dominant land use method, especially in urbanized areas with complex land covers. (3) The generalized Green–Ampt model is developed to simulate infiltration and infiltration excess during heavy rains to save computation time. (4) The infiltration trenches are simulated in the model, which makes it possible to evaluate their effect on the hydrological cycle. MODEL DEVELOPMENT Model structure The diagram of the model structure within a grid cell utilized in this study is shown in Figure 1a. To consider the subgrid heterogeneity of land use, the mosaic method is used which reflects composition of different land uses within a grid cell. The areal average of water and heat fluxes from all land uses in a grid cell produces the averaged fluxes in the grid cell. Land use is at first divided into three groups, namely a water body group, a soil–vegetation group and an impervious area group. The soil–vegetation group is further classified into bare soil, tall vegetation (forest or urban trees) and short vegetation (grass or crops). The impervious area group consists of impervious urban cover and urban canopy. For the soil–vegetation group, nine vertical layers, namely an interception layer, a depression layer, three upper soil layers, a transition layer, an unconfined aquifer and two confined aquifers, are included in the model structure. The energy balance of each land use is also analysed. The interaction of radiation between soil and vegetation is considered by use of the fraction of transmitted short-wave radiation of vegetation, whereas the interaction between urban cover and urban canopy is considered by using the sky view factor of urban cover. Copyright 2001 John Wiley & Sons, Ltd. Hydrol. Process. 15, 2175–2194 (2001) DEVELOPMENT OF WEP 2177 (a) Precipitation Shortwave Longwave radiation radiation Water Body Soil-Vegetation Impervious Group Group Area Group Anthropogenic Interception Transpiration energy source Layer heat Surface Depression fluxes runoff τ Layer Top Soil Layer Evaporation Suction Water use heat diffusion Infiltration 2nd Soil Layer leakage Pumped fluxes runoff ground Subsurface 3rd Soil Layer water Recharge Transition Layer Exchange by Flow Unconfined Aquifer Flow in seepage or out outflow Aquitard 1 Flow in Confined Aquifer 1 Percolation 1 Flow out Aquitard 2 Flow in Percolation 2 Flow Confined Aquifer 2 out (b) Multi-layered Groundwater Flow 1D River Flow j+1 j Lateral inflow j−1 Sub-Watershed Tributary Main River i−1i i+1 Figure 1. The structure of the proposed model : (a) vertical structure within a grid cell and (b) horizontal structure Copyright 2001 John Wiley & Sons, Ltd. Hydrol. Process. 15, 2175–2194 (2001) 2178 Y. JIA ET AL. The diagram of the model horizontal structure within a watershed is shown in Figure 1b. River flow routing is conducted for every tributary and a
Details
-
File Typepdf
-
Upload Time-
-
Content LanguagesEnglish
-
Upload UserAnonymous/Not logged-in
-
File Pages20 Page
-
File Size-