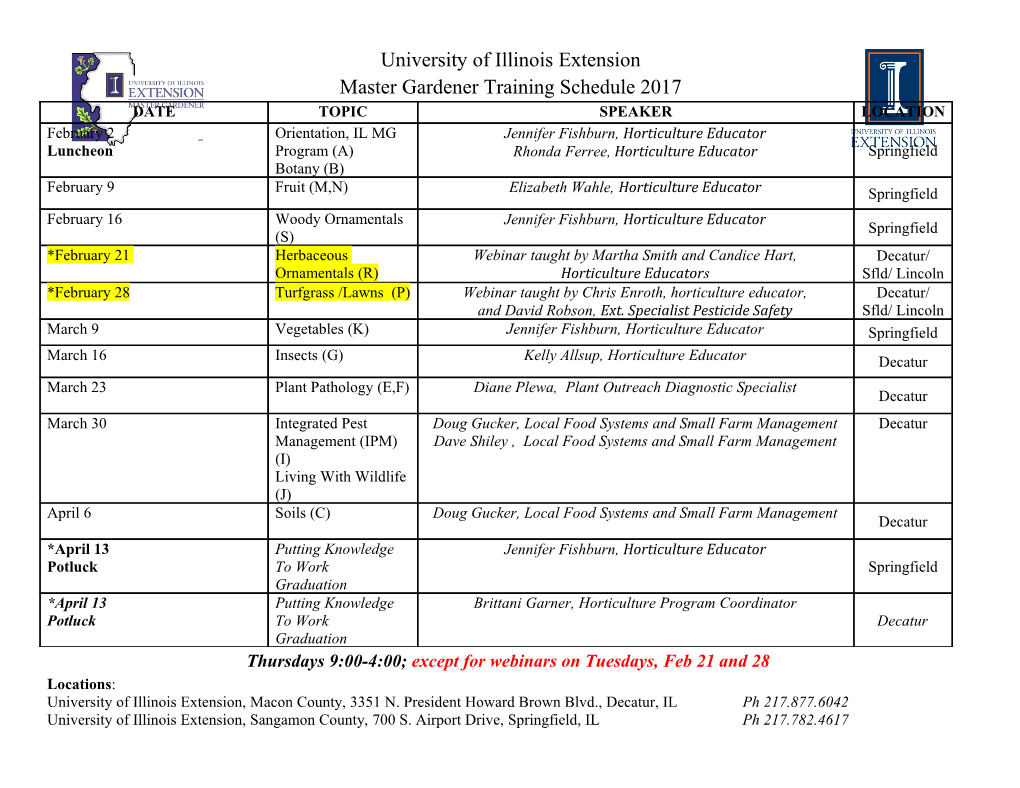
Geology, published online on 4 April 2013 as doi:10.1130/G33796.1 Intermediate-depth earthquakes facilitated by eclogitization-related stresses Junichi Nakajima1*, Naoki Uchida1, Takahiro Shiina1, Akira Hasegawa1, Bradley R. Hacker2, and Stephen H. Kirby3 1Research Center for Prediction of Earthquakes and Volcanic Eruptions, Graduate School of Science, Tohoku University, Sendai 980-8578, Japan 2Earth Research Institute, University of California–Santa Barbara, Santa Barbara, California 93106-9630, USA 3U.S. Geological Survey, Menlo Park, California 94025, USA ABSTRACT skipping of waveforms due to polarity reversal, Eclogitization of the basaltic and gabbroic layer in the oceanic crust involves a volume we calculated traveltime differences indepen- reduction of 10%–15%. One consequence of the negative volume change is the formation of dently for the reverse- and normal-fault groups. a paired stress fi eld as a result of strain compatibility across the reaction front. Here we use We applied hypoDD to the calculated differen- waveform analysis of a tiny seismic cluster in the lower crust of the downgoing Pacifi c plate tial traveltime data, and determined the relative and reveal new evidence in favor of this mechanism: tensional earthquakes lying 1 km above locations of the earthquakes in the reverse- and compressional earthquakes, and earthquakes with highly similar waveforms lying on well- normal-fault groups with the one-dimensional defi ned planes with complementary rupture areas. The tensional stress is interpreted to be velocity model (Ueno et al., 2002). caused by the dimensional mismatch between crust transformed to eclogite and underlying The relative locations of the reverse-fault untransformed crust, and the earthquakes are probably facilitated by reactivation of fossil earthquakes with respect to the normal-fault faults extant in the subducting plate. These observations provide seismic evidence for the role earthquakes were determined using S–P time of volume change–related stresses and, possibly, fl uid-related embrittlement as viable pro- differences. The polarity of waveform was cesses for nucleating earthquakes in downgoing oceanic lithosphere. reversed for the normal-fault earthquakes, and their coherencies with the reverse-fault earth- INTRODUCTION show that the cluster is located in the lowermost quakes were calculated in the same manner as Generation of intermediate-depth earth- part of the oceanic crust (Fig. 1B) and concen- for the earthquake classifi cation. Then, we iden- quakes is an ongoing enigma because high litho- trated in a volume no more than 2 km × 2 km × tifi ed the earthquake pair with the most-similar static pressures render ordinary dry frictional 5 km (Figs. 1C and 1D). The earthquakes in the waveform (an average coherency of 0.92) failure unlikely. Earthquakes in subducting oce- cluster have occurred not in swarms but fairly (Fig. DR2). For this pair, we calculated S–P anic crust have been linked to the transformation evenly over time (Fig. 1E). time differences at 14 common stations based of basalt to eclogite and concomitant dehydra- We selected 106 earthquakes (M ≥ 2) in the on waveform similarity, and determined the tion (e.g., Kirby et al., 1996; Preston et al., 2003; period from January 2003 to February 2011, and relative locations of the two earthquakes using Hacker et al., 2003). A major, untested predic- classifi ed the earthquakes into subgroups on the the S–P time differences. Finally, we shifted tion of this hypothesis is, however, that the large basis of waveform similarity. A time window of the other earthquakes with respect to that earth- (10%–15%) volume reduction during eclogite 30 s was set for the vertical component, begin- quake pair. formation should produce a paired stress regime ning 3 s before the onset of the P wave, and To estimate the extent of source areas, we along the eclogitization reaction front in which coherency was calculated using the cross spec- determined the source radii of the 30 earth- tension is underlain by compression (Hacker, tral method (Poupinet et al., 1984) for each pair quakes from corner frequencies. Corner fre- 1996; Kirby et al., 1996). This study presents of earthquakes in a frequency band of 2–12 Hz. quencies were estimated by applying the spectral the fi rst seismological evidence of such a paired When the calculated coherencies were larger ratio method to P-wave amplitude spectra for a stress fi eld associated with eclogite formation in than 0.9 at three or more stations, we regarded tapered 2.0 s time window of the vertical com- the oceanic crust. the earthquake pair as part of the same group. In ponent (e.g., Frankel and Wennerberg, 1989) this manner, we categorized 30 earthquakes into (Fig. DR3). We used an omega-square source DATA ANALYSIS nine groups with similar waveforms (Fig. 1F; model (Brune, 1970) to model the spectral The Pacifi c plate beneath northeastern Japan Fig. DR1 in the GSA Data Repository1): four ratio stacked for the available station pairs. As has a well-developed double seismic zone in groups (R1–R4) of 19 reverse-fault earthquakes a result, we obtained the corner frequencies of which earthquakes with downdip compression and fi ve groups (N1–N5) of 11 normal-fault eight earthquakes. The source radii of the eight dominate the upper seismic zone within the earthquakes. earthquakes were calculated using the circular crust, and earthquakes with downdip tension For precise relative earthquake relocations, crack model (Sato and Hirasawa, 1973). For the dominate the lower seismic zone 30–40 km traveltime differences of the 30 earthquakes 22 earthquakes without the estimates of cor- deeper within the mantle (e.g., Hasegawa et were calculated using the cross spectral method ner frequencies, we calculated the source radii al., 1978). Somewhat-isolated seismic clusters for both P and S waves. A time window of 3 s from the average stress drop (7 MPa) using the are observed locally in the upper seismic zone was set 0.5 s before the onset of each wave, and formula of Eshelby (1975). The radii of source at a depth of ~150 km (red and pink arrows in delay times were calculated from the phases of areas thus calculated range from 43 m to 216 m Fig. 1A). We investigate seismic features of the cross spectra in a frequency band of 2–12 Hz with stress drops of 3.5–33.3 MPa. Source most-active isolated seismic cluster (red arrow) with a coherency of >0.8. To avoid half-cycle parameters obtained for the 30 earthquakes are using high-quality waveform data. Hypocenters listed in Table DR1 in the Data Repository. of the cluster relocated by the double-difference method (hypoDD) (Waldhauser and Ellsworth, 1GSA Data Repository item 2013179, Figures RESULTS AND DISCUSSION DR1–DR3, and Table DR1, is available online at www 2000) with catalog-derived differential data .geosociety.org/pubs/ft2013.htm, or on request from The relocated hypocenters show important [email protected] or Documents Secretary, GSA, features in terms of earthquake-generating stress *E-mail: [email protected]. P.O. Box 9140, Boulder, CO 80301, USA. and seismic rupture areas. (1) The normal-fault GEOLOGY, June 2013; v. 41; no. 6; p. 1–4; Data Repository item 2013179 | doi:10.1130/G33796.1 | Published online XX Month 2013 GEOLOGY© 2013 Geological | June Society 2013 | ofwww.gsapubs.org America. For permission to copy, contact Copyright Permissions, GSA, or [email protected]. 1 Geology, published online on 4 April 2013 as doi:10.1130/G33796.1 Longitude (E) Longitude (E) 2001), some special process must produce local 138.5° 139° 139.5° 140° 140.5° 141° 141.5° 139.2° 139.24° 139.28° 139.32° 39° 37.96° tension. What process can generate earthquakes R1 N1 A C R2 N2 with local tension in proximity to compressional R3 N3 150 125 R4 N4 earthquakes and produce clusters of seismic N5 175 km events on well-defi ned and separate planes? 38.5° Y Pacific 37.92° Progressive eclogitization of the oceanic plate ′ crust of subducting slabs is interpreted from Latitude (N) Y X gradual increases in seismic velocities and 38° TU.SBT 100 the disappearance of seismic activity (e.g., 75 37.88° 2 km Helffrich, 1996; Abers, 2005; Kita et al., 2006). Latitude (N) The upper, basaltic layer of the crust trans- N.MRMH X’ Y Y′ 37.5° forms to eclogite more easily because of fi ner D 150 grain size, increased alteration, and higher tem- seismic station volcano plate interface peratures (e.g., Hacker, 1996); eclogitization of 37° XX′ basalt beneath northeastern Japan is interpreted 100 B 154 to occur at depths of 70–130 km (Igarashi et al., 120 2001; Kita et al., 2006). Transformation of the mantle Depth (km) Moho lower, gabbroic layer to eclogite is, however, 140 wedge plate interface Pacific Plate 158 retarded because of coarser grain size, substan- 160 tially less alteration, and lower temperatures 0246 810 180 Distance (km) (e.g., Hacker, 1996); because of this, eclogitiza- Depth (km) 4 tion likely progresses from the top of the crust 200 Moho 80 100 120 140 160 E downward (Fig. 4). A low-velocity layer at the depth of hypocenters (km) 3 220 04080120 160 200 top of the Pacifi c slab suggests that eclogitiza- 2 Distance (km) tion of the gabbroic layer is incomplete even Magnitude 1 at depths down to 150 km (Matsuzawa et al., 0 1986) where the seismic cluster described here 2004 2006 2008 2010 Year is located. F TU.SBT Vertical component Band-pass filter : 2-12 Hz One possibility to explain the observed seis- Group R2 mic cluster is that the eclogitic and gabbroic 101531125 M2.3 layers in the slab have different strengths and 083321938 M2.2 respond differently to the slab stress state.
Details
-
File Typepdf
-
Upload Time-
-
Content LanguagesEnglish
-
Upload UserAnonymous/Not logged-in
-
File Pages4 Page
-
File Size-