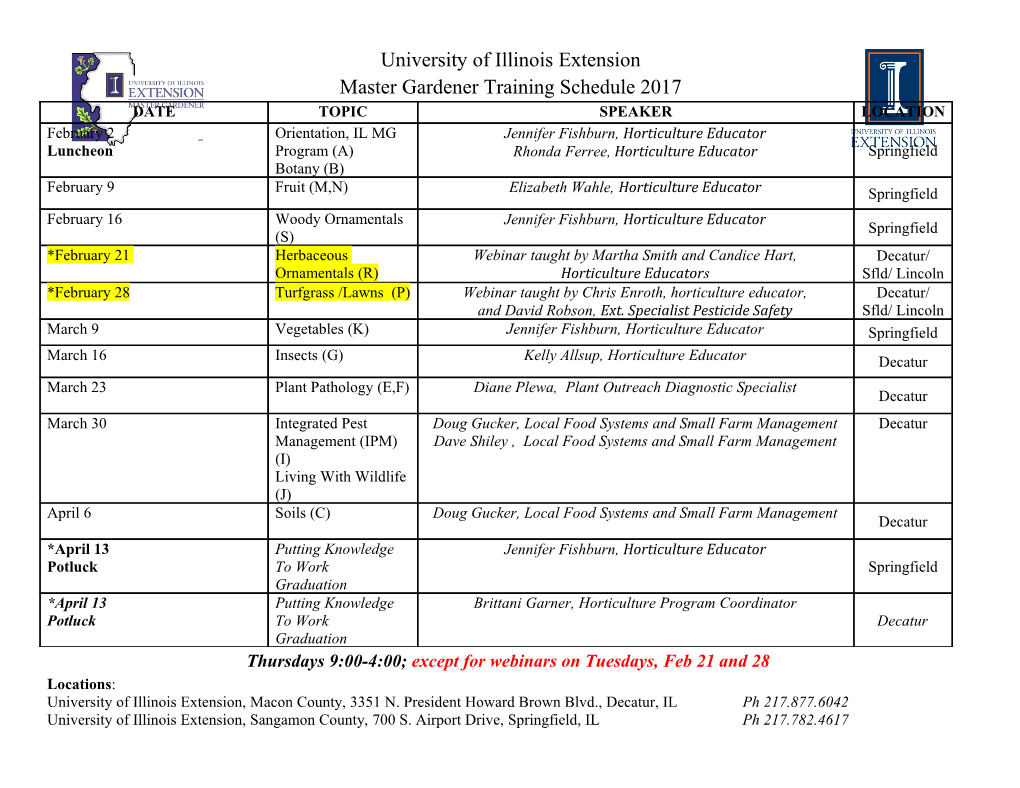
letters to nature more axisymmetric. Also, the magnetic field that penetrates the 17. Dormy, E., Valet, J. P. & Courtillot, V. Numerical models of the geodynamo and observational conducting solid inner core can only vary on the much longer constraints. Geochem. Geophys. Geosyst. 1, 2000GC000062 (2000). 18. Grote, E., Busse, F. H. & Tilgner, A. Regular and chaotic spherical dynamos. Phys. Earth Planet. Inter. magnetic diffusion timescale rather than the shorter timescale of 117, 259–272 (2000). fluid motions in the outer core. This effectively anchors the 19. Grote, E. & Busse, F. H. Hemispherical dynamos generated by convection in rotating spherical shells. magnetic field and stabilizes it against the rapid fluctuations in Phys. Rev. E 62, 4457–4460 (2000). 20. Kutzner, C. & Christensen, U. R. From stable dipolar towards reversing numerical dynamos. Phys. the outer core, allowing the field to maintain a more stable dipole. Earth Planet. Inter. 131, 29–45 (2002). If the region interior to the convecting shell is fluid instead of 21. Ishihara, N. & Kida, S. Equatorial magnetic dipole field intensification by convection vortices in a solid, then it can respond to electromagnetic stress through fluid rotating spherical shell. Fluid Dyn. Res. 31, 253–274 (2002). motion and is not constrained by the stabilizing effects of a solid 22. Aubert, J. & Wicht, J. Axial versus equatorial dipolar dynamo models with implications for planetary magnetic fields. Earth Planet. Sci. Lett. (in the press). conducting core. As the fluid inner region is not in solid-body 23. Roberts, P. H. & Glatzmaier, G. A. The geodynamo, past, present and future. Geophys. Astrophys. Fluid rotation, the axisymmetrizing effect of strong differential rotation is Dynam. 94, 47–84 (2001). suppressed. Also, the magnetic field that penetrates the inner region 24. Hollerbach, R. & Jones, C. A. Influence of the Earth’s inner core on geomagnetic fluctuations and can vary by moving the fluid instead of only through magnetic reversals. Nature 365, 541–543 (1993). 25. Wicht, J. Inner core conductivity in numerical dynamo simulations. Phys. Earth Planet. Inter. 132, diffusion. This reduction in stability, combined with the smaller 281–302 (2002). length scales of the thin shell, promote the creation of higher- 26. Marley, M., Gomez, P. & Podolak, M. Monte Carlo interior models for Uranus and Neptune. degree, non-axisymmetric structure in our numerical models with J. Geophys. Res. 100, 23349–23353 (1995). stably stratified fluid interiors. 27. Zhang, K. K. & Schubert, G. Teleconvection: remotely driven thermal convection in rotating stratified spherical layers. Science 290, 1944–1947 (2000). As the stabilizing effects of the inner core are a result of its solid 28. Jones, C. A., Longbottom, A. W. & Hollerbach, R. A self-consistent convection driven geodynamo state and conductivity, our theory predicts that a dynamo operating model, using a mean field approximation. Phys. Earth Planet. Inter. 92, 119–141 (1995). in a thin shell surrounding an electrically insulating solid inner core 29. Merrill, R. T., McElhinny, M. W. & McFadden, P. L. The Magnetic Field of the Earth 31 (Academic, San would also produce non-dipolar, non-axisymmetric fields. We have Diego, 1996). 30. Holme, R. & Bloxham, J. The magnetic fields of Uranus and Neptune: methods and models. J. Geophys. created numerical dynamos operating in a thin shell (similar radius Res. 101, 2177–2200 (1996). to our convecting shell surrounding the stably stratified interior) surrounding a large solid inner core with a much smaller electrical Acknowledgements We thank D. Stevenson for comments on the manuscript. This work was conductivity than the outer core (inner to outer core conductivity supported by NSERC and NSF. ratios of 0.1–0.0001), and found that they do produce non-dipolar, non-axisymmetric magnetic fields, as predicted. Competing interests statement The authors declare that they have no competing financial interests. Future missions to Uranus and Neptune to study further these planets’ magnetic fields and internal properties could determine the Correspondence and requests for materials should be addressed to S.S. validity of our model. More accurate surface power spectra extend- ([email protected]). ing out to higher degree and order could be produced with magnetic-field measurements acquired closer to the planets with more global spatial distribution. These spectra could then be compared to our numerical model to assess the model’s validity. The evolution in time of the magnetic fields could also be compared .............................................................. to our numerical model by examining the changes in the fields since the Voyager II observations. Although no missions to Uranus Observation of entanglement and Neptune are currently planned, the upcoming data from MESSENGER and Cassini (on the magnetic fields of Mercury and between a single trapped atom Saturn, respectively) will provide tests of the implication of our results: convective-region geometry is an important factor in and a single photon determining magnetic-field morphology, albeit in a different con- text from this study. A B. B. Blinov, D. L. Moehring, L.- M. Duan & C. Monroe Received 9 October 2003; accepted 28 January 2004; doi:10.1038/nature02376. FOCUS Center and University of Michigan Department of Physics, Ann Arbor, 1. Ness, N. F. et al. Magnetic fields at Uranus. Science 233, 85–89 (1986). Michigan 48109-1120, USA 2. Ness, N. F. et al. Magnetic fields at Neptune. Science 246, 1473–1478 (1989). ............................................................................................................................................................................. 3. Connerney, J. E. P., Acuna, M. H. & Ness, N. F. The magnetic field of Uranus. J. Geophys. Res. 92, 15329–15336 (1987). An outstanding goal in quantum information science is the 4. Connerney, J. E. P., Acuna, M. H. & Ness, N. F. The magnetic field of Neptune. J. Geophys. Res. 96, faithful mapping of quantum information between a stable 19023–19042 (1991). quantum memory and a reliable quantum communication chan- 5. Connerney, J. E. P. Magnetic fields of the outer planets. J. Geophys. Res. 98, 18659–18679 (1993). nel1. This would allow, for example, quantum communication 6. Russell, C. T. Magnetic fields of the terrestrial planets. J. Geophys. Res. 98, 18681–18695 (1993). 2 3 7. Ruzmaikin, A. A. & Starchenko, S. V. On the origin of Uranus and Neptune magnetic fields. Icarus 93, over remote distances , quantum teleportation of matter and 82–87 (1991). distributed quantum computing over a ‘quantum internet’. 8. Podolak, M., Hubbard, W. B. & Stevenson, D. J. in Uranus (eds Bergstralh, J. T., Miner, E. D. & Because quantum states cannot in general be copied, quantum Matthews, M. S.) 29–61 (Univ. Arizona Press, Tucson, 1991). 9. Hubbard, W. B., Podolak, M. & Stevenson, D. J. in Neptune and Triton (ed. Cruikshank, D. P.) 109–138 information can only be distributed in these and other appli- (Univ. Arizona Press, Tucson, 1995). cations by entangling the quantum memory with the communi- 10. Holme, R. Three-dimensional kinematic dynamos with equatorial symmetry: application to the cation channel. Here we report quantum entanglement between magnetic fields of Uranus and Neptune. Phys. Earth Planet. Inter. 102, 105–122 (1997). an ideal quantum memory—represented by a single trapped 11. Hubbard, W. B. et al. Interior structure of Neptune—comparison with Uranus. Science 253, 648–651 111 1 (1991). Cd ion—and an ideal quantum communication channel, 12. Nellis, W. J. et al. The nature of the interior of Uranus based on studies of planetary ices at high provided by a single photon that is emitted spontaneously dynamic pressure. Science 240, 779–781 (1988). from the ion. Appropriate coincidence measurements between 13. Kuang, W. & Bloxham, J. An earth-like numerical dynamo model. Nature 389, 371–374 (1997). 14. Kuang, W. J. & Bloxham, J. Numerical modelling of magnetohydrodynamic convection in a the quantum states of the photon polarization and the trapped rapidly rotating spherical shell: weak and strong field dynamo action. J. Comput. Phys. 153, 51–81 ion memory are used to verify their entanglement directly. Our (1999). direct observation of entanglement between stationary and ‘fly- 15. Glatzmaier, G. A. & Roberts, P. H. A 3-dimensional convective dynamo solution with rotating and ing’ qubits4 is accomplished without using cavity quantum finitely conducting inner core and mantle. Phys. Earth Planet. Inter. 91, 63–75 (1995). 5–7 16. Glatzmaier, G. A. & Roberts, P. H. A 3-dimensional self-consistent computer simulation of a electrodynamic techniques or prepared non-classical light 3 geomagnetic field reversal. Nature 377, 203–209 (1995). sources . We envision that this source of entanglement may be 153 NATURE | VOL 428 | 11 MARCH 2004 | www.nature.com/nature © 2004 Nature Publishing Group letters to nature used for a variety of quantum communication protocols2,8 and for reminiscent of the production of entangled photon pairs through seeding large-scale entangled states of trapped ion qubits for spontaneous optical parametric down-conversion3,butinthe scalable quantum computing9. current system one of the two daughter qubits resides within a Atom–photon entanglement has been implicit in many previous trapped atomic ion—perhaps the most reliable of all qubit mem- experimental systems, from early measurements of Bell inequality ories18. When accompanied by conventional quantum gates violations in atomic cascade
Details
-
File Typepdf
-
Upload Time-
-
Content LanguagesEnglish
-
Upload UserAnonymous/Not logged-in
-
File Pages5 Page
-
File Size-