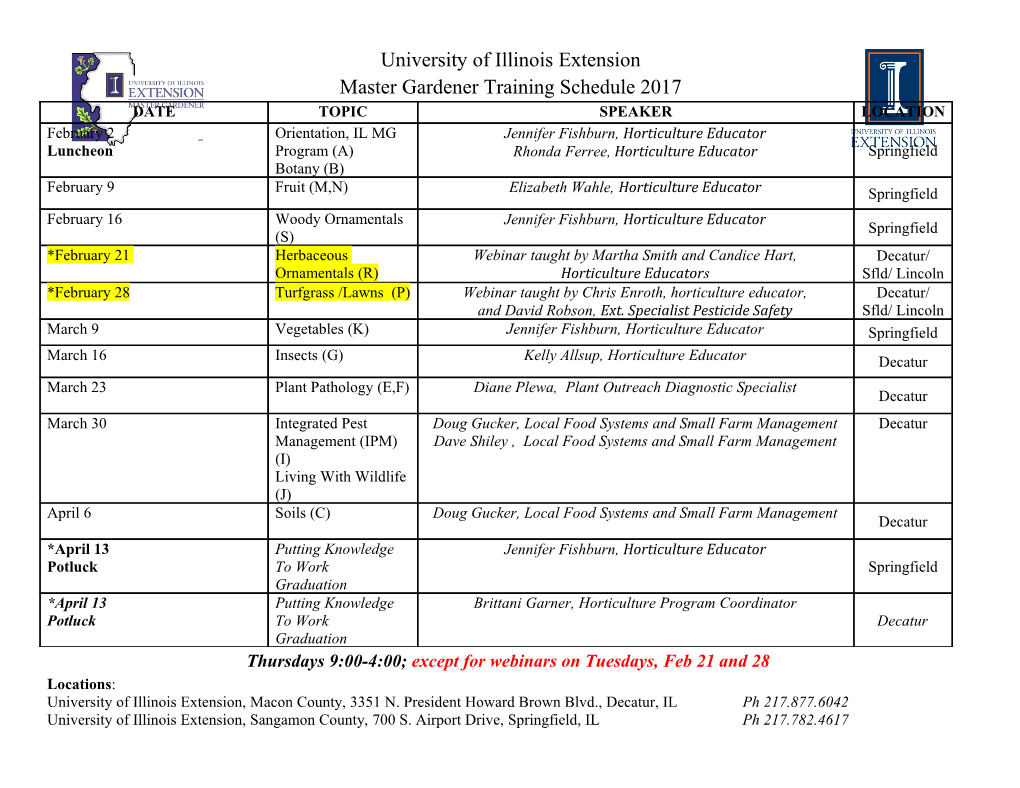
Hypothalamic ATP-sensitive K؉ Channels Play a Key Role in Sensing Hypoglycemia and Triggering Counterregulatory Epinephrine and Glucagon Responses Mark L. Evans, Rory J. McCrimmon, Daniel E. Flanagan, Tara Keshavarz, Xiaoning Fan, Ewan C. McNay, Ralph J. Jacob, and Robert S. Sherwin It has been postulated that specialized glucose-sensing neurons in the ventromedial hypothalamus (VMH) are able to detect falling blood glucose and trigger the he benefits of lowering average blood glucose release of counterregulatory hormones during hypogly- levels in diabetes to reduce the risk of long-term cemia. The molecular mechanisms used by glucose-sens- complications are well established. In clinical ing neurons are uncertain but may involve cell surface practice, the degree to which this can be ؉ T ATP-sensitive K channels (KATP channels) analogous achieved is often limited by the increased risk of hypogly- to those of the pancreatic -cell. We examined cemia that accompanies intensified glucose-lowering reg- whether the delivery of sulfonylureas directly into the imens (1,2). When healthy, blood glucose levels are brain to close KATP channels would modulate counter- normally maintained within relatively narrow limits. A fall regulatory hormone responses to either brain gluco- in blood glucose is rapidly detected and a series of penia (using intracerebroventricular 5-thioglucose) compensatory homeostatic responses are triggered that or systemic hypoglycemia in awake chronically cathe- tend to prevent or limit hypoglycemia and to restore terized rats. The closure of brain KATP channels by global intracerebroventricular perfusion of sulfonyl- euglycemia. These responses include secretion of the urea (120 ng/min glibenclamide or 2.7 g/min tolbut- counterregulatory hormones glucagon and epinephrine, amide) suppressed counterregulatory (epinephrine which promote endogenous glucose production and limit and glucagon) responses to brain glucopenia and/or tissue utilization of glucose and the generation of typical systemic hypoglycemia (2.8 mmol/l glucose clamp). warning symptoms. These protective responses against Local VMH microinjection of a small dose of gliben- hypoglycemia are disrupted in diabetes. Within 5 years of clamide (0.1% of the intracerebroventricular dose) diagnosis, almost all patients with type 1 diabetes will also suppressed hormonal responses to systemic hypo- develop defective secretion of the counterregulatory hor- glycemia. We conclude that hypothalamic KATP channel mone glucagon during hypoglycemia (3). This leaves epi- activity plays an important role in modulating the hor- nephrine as the major hormonal counterregulatory monal counterregulatory responses triggered by de- defense against low blood glucose. However, a significant creases in blood glucose. Our data suggest that closing number of patients will also develop additional deficien- of K channels in the VMH (much like the -cell) ATP cies in epinephrine and other neurohumoral responses to impairs defense mechanisms against glucose depriva- hypoglycemia associated with the loss of symptomatic tion and therefore could contribute to defects in glu- cose counterregulation. Diabetes 53:2542–2551, 2004 awareness of hypoglycemia (3). The combination of im- paired counterregulation and hypoglycemia unawareness significantly increases the risk of suffering severe episodes of hypoglycemia (4,5). To trigger protective counterregulatory neurohumoral responses, hypoglycemia must first be detected. Although low blood glucose may be detected, at least in part, by peripheral sensors (6–9), much evidence suggests that From the Diabetes Endocrine Research Center, Yale School of Medicine, New Haven, Connecticut. hypoglycemia is sensed predominantly by the brain (10– Address correspondence and reprint requests to Professor Robert Sherwin, 13) by glucose-sensing neurons in the ventromedial hypo- Diabetes Endocrine Research Center, Fitkin 1, Yale School of Medicine, 333 thalamus (VMH), with ventromedial and arcuate nuclei Cedar St., New Haven, CT 06520. E-mail: [email protected]. M.L.E. and R.J.M. contributed equally to this study. probably playing a key role (14–16). Glucose-sensing M.L.E. is currently affiliated with the Department of Medicine, Adden- neurons may be either stimulated (glucose excited) or brookes Hospital, University of Cambridge, Cambridge, U.K. Received for publication 29 March 2004 and accepted in revised form 13 inhibited (glucose inhibited) by a rise in glucose (17). The July 2004. mechanisms used by specialized glucose excited and 5TG, 5-thioglucose; aECF, artificial extracellular fluid; KATP channel, ATP- inhibited neurons to sense changes in glucose remain sensitive Kϩ channel; KIR, pore-forming subunit; SUR, sulfonylurea receptor subunit; VMH, ventromedial hypothalamus. undetermined, but recent in vitro evidence suggests that © 2004 by the American Diabetes Association. there may be parallels with pancreatic -cell glucose 2542 DIABETES, VOL. 53, OCTOBER 2004 M.L. EVANS AND ASSOCIATES sensing (18,19). In the classical pathway of -cell glucose bolus of 5TG. Control animals received vehicle infusions (5% DMSO/aECF) for sensing, a fall in blood glucose leads, via a fall in intracel- 120 min (n ϭ 5) but were otherwise treated identically. Study 3: effects of intracerebroventricular glibenclamide on responses lular ATP, to the opening of KATP channels and thus cell to systemic hypoglycemia. Surgeries and preparations were performed as membrane hyperpolarization (leading to decreased insulin described above for study 1. A continuous glibenclamide (120 ng/min, n ϭ 9)  ϭ secretion). KATP channels thus allow -cells to transduce or vehicle (n 10) infusion was commenced as in study 1 and continued the metabolic signal of altered ambient glucose levels into throughout the study. After 60 min of euglycemia, a primed-continuous intravascular infusion of 10 mU ⅐ kgϪ1 ⅐ minϪ1 regular insulin (Humulin R; Eli changes in cell membrane electrical charge, eventually Lilly, Indianapolis, IN) was started together with a variable infusion of 10% leading to altered insulin secretion. KATP channels are dextrose. To maintain euglycemia for an additional 60 min, the dextrose present in brain (20), and electrophysiological studies of infusion was adjusted in response to 5- to 10-min bench-top measurements of rat brain slices have demonstrated that sulfonylureas plasma glucose. At this time, the dextrose infusion rate was decreased and the (drugs that act to inhibit and in turn close K channels) plasma glucose allowed to fall rapidly to a target value of 2.8 mmol/l. The ATP dextrose infusion was then adjusted to maintain this level for an additional 45 can stimulate the firing of glucose-excited neurons min. In addition to monitoring plasma glucose, additional volumes of blood (18,21,22). were drawn 15 min (for glucagon) and 45 min (for epinephrine, glucagon, Our hypothesis was that hypothalamic KATP channels insulin, and C-peptide) after the onset of hypoglycemia. play a key role in the sensing of hypoglycemia in brain. We Study 4: effects of VMH glibenclamide on responses to systemic hypo- glycemia. The animals underwent surgery for placement of vascular catheters examined this in an in vivo model, measuring the effects of as described above. In addition, under the same anesthesia, guide cannulae for direct delivery of agents acting on KATP channels into rat microinjectors were placed bilaterally into the VMH (coordinates from brain during both brain glucopenia and controlled sys- bregma Ϫ2.6 mm anteroposterior, Ϯ3.8 mm lateral, 9.5 mm at 20° dorsoven- temic hypoglycemia. Our results support a key role for tral). Animals were studied 7 days after an overnight fast (16 h). Microinjec- brain K channels in the detection of low blood glucose tion cannulae (designed to extend 1 mm beyond the guide cannula tip) were ATP inserted through the guide cannulae and 12.4 ng glibenclamide injected and the triggering of counterregulatory hormonal re- bilaterally (0.25 l in volume to each side). The total dose of glibenclamide sponses to hypoglycemia. delivered into the VMH represented ϳ0.1% of the dose delivered into the brain by intracerebroventricular infusion in studies 1 and 3 above. Fifteen minutes after the VMH injections, baseline blood samples were taken and a 10-mU ⅐ RESEARCH DESIGN AND METHODS kgϪ1 ⅐ minϪ1 insulin infusion was started together with a variable infusion of Male Sprague-Dawley rats were purchased from Charles River Laboratories 10% dextrose, as described in study 3. On this occasion, plasma glucose was (Wilmington, MA). The animals were housed in the Yale Animal Care Facility lowered in two steps, aiming for a plateau of 3.3 mmol/l between 30 and 60 in an environmentally controlled room with a 12-h light/dark cycle and fed a min, with a second step of 2.5 mmol/l between 80 and 110 min. standard ad libitum rat diet comprised of 22% protein, 5% fat, and 51% Lab measurements. Epinephrine was measured by high-performance liquid carbohydrate (Agway Prolab 3000; Agway, Syracuse, NY). The protocol was chromatography with electrochemical detection (ESA, Acton, MA) and gluca- reviewed and approved by the Yale Animal Care and Use Committee. gon, C-peptide, and insulin by radioimmunoassay (Linco, St. Charles, MO). Study 1: effects of intracerebroventricular glibenclamide on responses Statistical analysis. Epinephrine responses in studies 1–3 and insulin/C- to brain glucopenia. Rats were anesthetized with intraperitoneal ketamine peptide data in study 2 were not normally distributed
Details
-
File Typepdf
-
Upload Time-
-
Content LanguagesEnglish
-
Upload UserAnonymous/Not logged-in
-
File Pages10 Page
-
File Size-