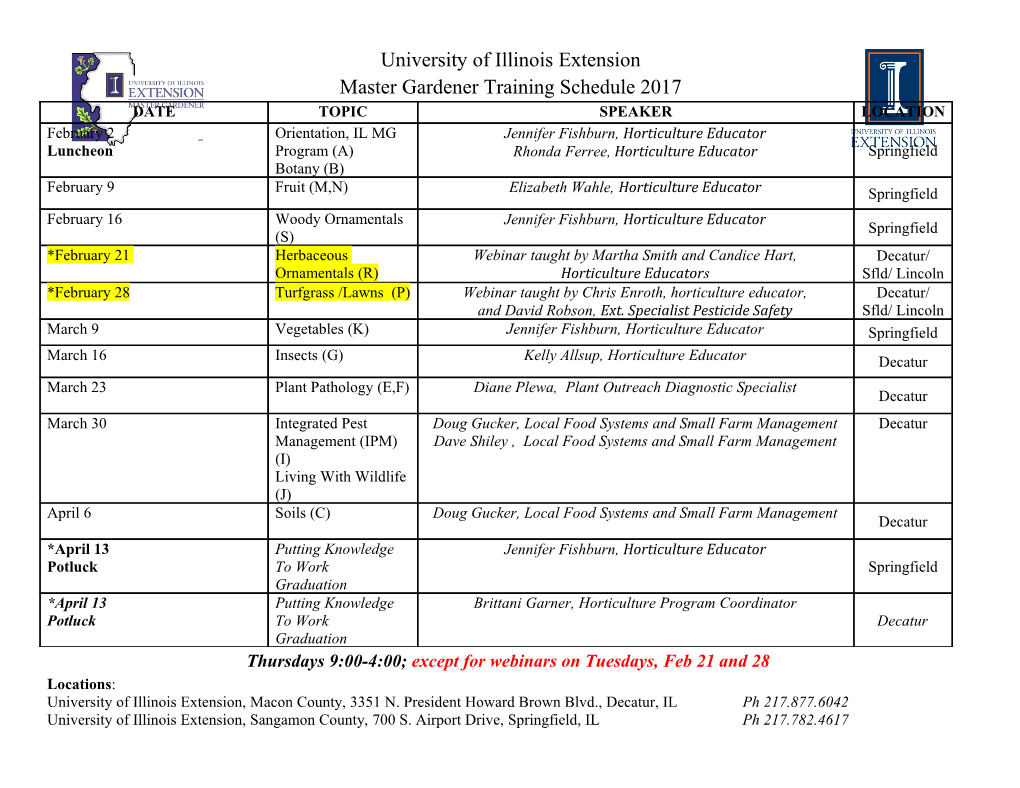
Energetic Molecules as Future Octane Boosters: Theoretical and Experimental Study Dissertation by Mohannad Ali Abdullah Al-Khodaier In Partial Fulfillment of the Requirements For the Degree of Doctor of Philosophy King Abdullah University of Science and Technology Thuwal, Kingdome of Saudi Arabia © June 2018 Mohannad Ali Abdullah Al-Khodaier All Rights Reserved 1 2 EXAMINATION COMMITTEE PAGE The dissertation of Mohannad Ali Abdullah Al-Khodaier is approved by the examination committee. Committee Chairperson: Prof. Mani S. Sarathy Committee Member: Prof. Kazahiro Takanabe, Prof. Luigi Cavallo, Prof. Mohammed Ali Morsy 3 ABSTRACT Energetic Molecules as Future Octane Boosters: Theoretical and Experimental Study Mohannad Ali Abdullah Al-Khodaier The utilization of energetic strained molecules may be one way to mitigate carbon emissions or better and more economical fuel blends. To investigate candidate molecules, limonene and dicyclopentadiene, both theoretical and experimental procedures were implemented here. Computational quantum chemistry methods were employed to determine the thermodynamic properties and kinetic parameters for the hydrogen-abstraction reactions of limonene by a hydrogen atom. Geometry optimization and energy calculation was conducted for all stable species and transition states using Gaussian 09. The rate constants of the H-abstraction reactions were calculated using conventional transition state theory, as implemented in ChemRate software. The obtained values were fitted over the temperature range of 298 – 2000 K to obtain the modified Arrhenius parameters. Increasing the anti-knock quality of gasoline fuels can enable higher efficiency in spark ignition engines. This study explores blending the anti-knock quality of dicyclopentadiene (DCPD, a by-product of ethylene production from naphtha cracking), with various gasoline fuels. The blends were tested in an ignition quality tester (IQT) and a modified cooperative fuel research (CFR) engine operating under homogenous charge compression ignition (HCCI) and knock limited spark advance (KLSA) conditions. Ethanol is widely used as a gasoline blending component in many markets, due to current fuel regulations. The test results of DCPD-gasoline blends were compared to those of ethanol-gasoline blends. 4 Furthermore, the anti-knock properties of dicyclopentadiene (DCPD) as an additive to primary reference fuels (PRF) and toluene primary reference fuels (TPRF) have been investigated. The research octane number (RON) and motor octane number (MON) were measured using cooperative fuels research (CFR) engine for four different fuel blends. Moreover, the ignition delay times of these mixtures were measured in a high-pressure shock tube at 40 bar and stoichiometric mixtures over a temperature range of [700-1200 K]. Ignition delay measurements were also conducted using rapid compression machine (RCM) at stoichiometric conditions and 20 bar. An ignition quality tester (IQT) compared ignition delay times of iso-octane and DCPD. Furthermore, a chemical kinetic auto-ignition model was designed to simulate the IDT experiments. 5 ACKNOWLEDGEMENTS My gratitude to almighty Allah for his blessings without which it would not have been possible to accomplish this work. Many people have assisted me during my years of research at KAUST; to them I offer my heartfelt thanks. First, I would like to express my deep gratitude to my parents for their prayers and support. I would also thank my dear wife for her support during my five years at KAUST. A special thank you is extended to Professor Mani Sarathy for his valuable help and guidance, and to thank my colleagues: Mariam El-Rachidi, Samah Mohammad, Nour Atef, Vijai Shankar Bhavani Shankar, Adamu Al-Fazazi, and Mohammad Al-Abbad. Lab Manager Issam Al-Khesho was both a best friend and mentor; I am very grateful for his help and support. Finally, I would like to thank everyone at CCRC and KAUST who had a role in making my experience there so enjoyable. 6 TABLE OF CONTENTS ABSTRACT……………………………………………………….……………………...3 ACKNOWLEDGEMENTS……………………………………………………………..5 TABLE OF CONTENTS……………………………………….………………………..6 LIST OF ABBREVIATIONS…………………………………………………………..10 LIST OF FIGURES……………………………………………..………………………11 LIST OF TABLES…………………………………………..…………………………..15 1. Introduction…………………………………………...………………………...17 1.1. Van der Waals strain…………………………………………...…………….17 1.1.1. Syn-pentane strain……………………………………………………..…18 1.1.2. Allylic strain……………………………………………..……………….19 1.1.3. 1,3-diaxial strain…………………………………………..……………...19 1.2. Torsional strain…………………………………………………………...….20 1.3. Angle strain…………………………………………………...……………...21 1.4. Measuring the energy content of molecules………………………………….24 1.4.1. Heat of formation……………………………….…………………….25 1.4.2. Heat of combustion………………………….………………………..26 1.5. Review of literature on high energy, organic, strained molecules…………………………………………..………………..………28 1.5.1. Patent literature survey………………………..………………………28 1.6. Literature survey………………………………………...…………………...39 7 1.6.1. Sourcing and selection………………………..……………………….39 1.6.2. Renewable strained molecule fuel components………………..……...47 1.6.3. Prediction and measurement of physical and chemical properties……………………………………………………………..48 1.7. High energy density molecules as fuel blending components………………...52 1.8. Numerical methodologies…………………………...……………………….53 1.8.1. Computational chemistry……………………………….…………….53 1.8.2. Chemical kinetic modelling…………………………..……………….59 1.9. Experimental methodologies……………………………..………………….62 1.9.1. Cooperative fuel research engine………...……………….…………..62 1.9.2. Ignition quality tester………………...………………………..………62 1.9.3. Shock tube………………...………...………………………..……….63 1.9.4. Rapid compression machine………...……………………..………….63 1.10. Thesis structure………………………………………………….……….64 1.11. References…………………………………………………….………….66 2. Evaluation of Anti-Knock Quality of Dicyclopentadiene-Gasoline/PRF and TPRF Blends …………………………...…………………….…………...…….70 2.1. Introduction………………………………………………………...………...70 2.2. Fuels………………………………………………………………………….75 2.2.1. Dicyclopentadiene…………..………………………………………..75 2.2.2. Fuel blends…………………………………………………………...79 2.3. Experimental.……………………………………………….………………..80 2.3.1. Ignition Delay Time Measurements in Ignition Quality Tester.............81 8 2.3.2. Cooperative fuel research (CFR) engine ……………………...............83 2.3.2.1. Homogenous Charge Compression Ignition (HCCI) Number……83 2.3.2.2. Knock Limited Spark Advance (KLSA)………………………….88 2.3.2.3. Research and motored octane numbers…………………………...89 2.3.3. Shock tube ignition delay measurements…………………..…………89 2.3.4. Rapid compression machine..………………………………………...91 2.3.5. Chemical kinetic simulations………………………………………...92 2.4. Results and discussion…...…………………………………………………...86 2.4.1. Derived RON (DRON) from IQT……………………….……………94 2.4.2. HCCI Numbers……………………………………………………….97 2.4.3. Knock limited spark advance (KLSA)………………………………101 2.4.4. Research and motored octane numbers……………………………...107 2.4.5. Rapid compression ignition results………………………………….110 2.4.6. Shock tube experimental and simulation results…………………….111 2.4.7. Homogenous charge compression ignition and ignition quality tester………………………………………………………………...114 2.5. HCCI and IQT Correlation………………….…………………....................118 2.6. Chemical Nature of Synergistic Blending…………………………………..119 2.7. Kinetic analysis……………………………………………………………..121 2.8. Conclusions…………………………………………………………………126 2.9. References…………………………………………………………………..130 3. Theoretical Study of Hydrogen Abstraction from Limonene by H atom…...135 3.1. Introduction………………………………………………………...……….135 9 3.2. Computational method……………………………………………………...138 3.3. Results and discussion………………………………………………………144 3.3.1. Thermodynamic data……………………………………...................145 3.3.2. High pressure rate rules…………………………………...................147 3.4. Comparison with literature………………………………………………….152 3.5. Conclusions……………………………………………………....................156 3.6. References…………………………………………………………………..158 4. Conclusions and Future Work………………………………..........................162 APPENDICES…………………………………………………………........................164 Appendix A………………………………………………………......................164 Appendix B………………………………………………………......................167 Appendix C………………………………………………………......................185 10 LIST OF ABBREVIATIONS DCPD dicyclopentadiene TOF time of flight IQT ignition quality tester GC gas chromatography CFR cooperative fuel research FID flame ionization detector HCCI homogenous charge compression ignition QMS quadrupole mass spectrometer KLSA knock limited spark advance GA group additivity PRF primary reference fuel RCM rapid compression machine TPRF toluene primary reference fuel IDT ignition delay time RON research octane number MTBE methyl tert-butyl ether MON motor octane number BTU British thermal unit RMSEC root mean square error of calibration DFT density function theory RMSEP root mean square error of prediction QSPR quantitative structure properly relationship TST transition state theory ASTM American society for testing and CFD computational fluid dynamics materials SI spark ignition PLS partial least squares LFER linear free-energy relationships SRR structure-reactivity relationships 11 LIST OF FIGURES Figure 1.1 Van der Waals Strain in 1,2-dibromocyclopropane…………………………...18 Figure 1.2. Syn-pentane strain……………………………………………………………18 Figure 1.3. Allylic strain……………………………………………………………….…19 Figure 1.4. 1,3-diaxial strain in 1,4-dibromocyclohexane………………………………..19 Figure 1.5. Conformations of butane in Newman projection format……………………...20 Figure
Details
-
File Typepdf
-
Upload Time-
-
Content LanguagesEnglish
-
Upload UserAnonymous/Not logged-in
-
File Pages185 Page
-
File Size-