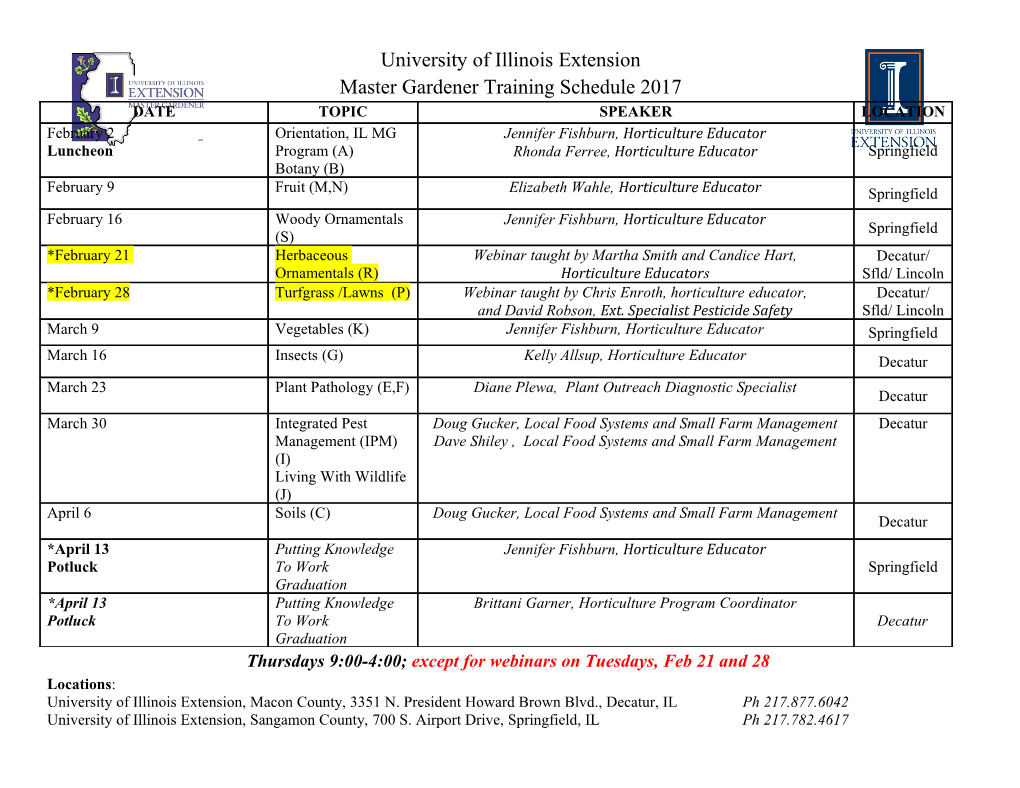
Complex Surfaces Andrew Kobin Spring 2019 Contents Contents Contents 0 Introduction 1 1 Complex Manifolds 2 1.1 Holomorphic Functions . .2 1.2 Complex Manifolds . .3 1.3 Dolbeault Cohomology . .6 1.4 K¨ahlerManifolds . .8 1.5 Analytic Varieties . 13 1.6 Divisors, Line Bundles and Sections . 15 1.7 Cohomology and Chern Classes . 21 1.8 Connections on Vector Bundles . 30 i 0 Introduction 0 Introduction These notes are from a course on 4-manifolds taught by Dr. Tom Mark at the University of Virginia in Spring 2019. I stuck around for the beginning of the course to take notes on complex manifolds, complex surfaces and surface classification since these topics are developed in parallel to the algebro-geometric theory of surfaces. The main reference for this course is Griffiths-Harris, Principles of Algebraic Geometry. 1 1 Complex Manifolds 1 Complex Manifolds 1.1 Holomorphic Functions Recall that a complex function f : C ! C is holomorphic if it satisfies the Cauchy-Riemann equations: @u @v @u @v = and = − @x @y @y @x where f = u + iv. To express this for functions of multiple complex variables, we can reinterpret this definition as follows. Set z = x + iy and define differentials dz = dx + i dy, dz¯ = dx − i dy and @f @f df = dx + dy: @x @y 1 i Then dx = 2 (dz + dz¯), dy = − 2 (dz − dz¯) and 1 @f @f 1 @f @f df = − i dz + + i dz¯ 2 @x @y 2 @x @y which suggests defining the following complex derivatives: @f 1 @f @f @f 1 @f @f = − i and = + i : @z 2 @x @y @z¯ 2 @x @y @f @f Then df = @z dz + @z¯ dz¯. Lemma 1.1.1. A complex function in one variable f = u + iv : C ! C is holomorphic if @f and only if @z¯ = 0. n For a multivariable function f : C ! C, write zj = xj + iyj, 1 ≤ j ≤ n, for the coordinates on Cn. Then n n n n X @f X @f X @f X @f df = dx + dy = dz + dz¯ @x j @y j @z j @z¯ j j=1 j j=1 j j=1 j j=1 j where @f and @f are defined in the same way as their single-variable counterparts above. @zj @z¯j n Definition. Let f : C ! C be a complex function in the variables zj = xj + iyj, 1 ≤ j ≤ n, and define n n X @f X @f @f = dz and @f¯ = dz¯ : @z j @z¯ j j=1 j j=1 j Then f is holomorphic if @f¯ = 0. Lemma 1.1.2. A function f : Cn ! C is holomorphic if and only if it satisfies the Cauchy- Riemann equations in each variable zj. n m Definition. More generally, f = (f1; : : : ; fm): C ! C is holomorphic if f1; : : : ; fm are all holomorphic. 2 1.2 Complex Manifolds 1 Complex Manifolds 1.2 Complex Manifolds Definition. A complex n-manifold is a Hausdorff topological space that is locally homeo- morphic to (an open subset of) Cn and has holomorphic transition functions. Explicitly, M is a complex manifold if it admits a complex atlas: a choice of open covering fUig of X n together with homeomorphisms 'i : Ui ! 'i(Ui) ⊆ C such that for each pair of overlapping charts Ui;Uj, the transition map −1 'ij := 'j ◦ 'i : 'i(Ui \ Uj) −! 'j(Ui \ Uj) and its inverse are holomorphic. A complex structure on M is the choice of such a complex atlas, up to holomorphic equivalence of charts, defined by a similar condition to the above. Let M be a complex n-manifold and fix a point p 2 M. Then in a chart U ⊆ M around p, the complex structure determines local parameters dx1; : : : ; dxn; dy1; : : : ; dyn. As in the previous section, there are also complex parameters dzj = dxj + i dyj and dz¯j = dxj − i dyj for 1 ≤ j ≤ n. Each of the dxj; dyj; dzj; dz¯j are R-linear functionals TpM ! R. Writing dzj = dxj + i dyj extends these to C-linear functionals TpM ! C (and likewise for dz¯j), so ∗ @ 1 @ @ @ 1 @ @ each dzj; dz¯j 2 T M ⊗ . Similarly, each = − i and = + i p R C @zj 2 @xj @yj @z¯j 2 @xj @yj ∗ are elements of the complexified tangent space TpM ⊗R C. Note that TpM ⊗ C and Tp ⊗ C are both 2n-dimensional complex vector spaces (so 4n-dimensional real vector spaces). The above functionals are cotangent vectors and can be evaluated on the above tangent vectors as follows: for 1 ≤ j; k ≤ n, @ 1 @ @ dzj = (dxj + i dyj) − i @zk 2 @xk @yk 1 @ @ @ @ = dxj + dyj + i dyj − dxj 2 @xk @yk @xk @yk 1 = [(δ + δ ) + i(0 − 0)] = δ : 2 ij ij ij @ @ @ n @ @ @ @ o Likewise, dz¯j = δij and dzj = dz¯j = 0. Thus ;:::; ; ;:::; @z¯k @z¯k @zk @z1 @zn @z¯1 @z¯n ∗ and fdz1; : : : ; dzn; dz¯1; : : : ; dz¯ng are dual bases for the vector spaces TpM ⊗ C and Tp M ⊗ C, respectively. As a consequence, there are several different notions of \tangent space" for a complex manifold: Real tangent space: the underlying real vector space of TpM, which has real dimen- sion 2n; C Complex tangent space: the complex vector space Tp M := TpM ⊗ C, which has complex dimension 2n; n on 0 @ C Holomorphic tangent space: the subspace TpM := Span @z of Tp M; C j j=1 n on 00 @ C Antiholomorphic tangent space: the subspace Tp M := Span @z¯ of Tp M. C j j=1 3 1.2 Complex Manifolds 1 Complex Manifolds ∗ C;∗ We also define the real and complex cotangent spaces Tp M and Tp M, as well as their 1;0 0 ∗ 0;1 00 ∗ holomorphic and antiholomorphic counterparts Tp M := (TpM) and Tp M := (Tp M) , respectively. Proposition 1.2.1. Let M be a complex manifold of dimension n and p 2 M. Then C 0 00 (1) Tp M = TpM ⊕ Tp M. C 0 00 (2) Complex conjugation acts C-linearly on Tp M and exchanges TpM and Tp M. C;∗ 1;0 0;1 (3) Tp M = Tp M ⊕ Tp M, and specifically we have 1;0 C;∗ 00 n Tp M = fα 2 Tp M j α ≡ 0 on Tp Mg = SpanCfdzjgj=1 0;1 C;∗ 0 n Tp M = fα 2 Tp M j α ≡ 0 on TpMg = SpanCfdz¯jgj=1: C;∗ 1;0 0;1 (4) Complex conjugation acts C-linearly on Tp M and exchanges Tp M and Tp M. Suppose M m and N n are complex manifolds with open subsets U ⊆ M; V ⊆ N, and f : U ! V is an arbitrary function. For 1 ≤ j ≤ m, write the coordinates on U as zj = xj + iyj and similarly, for 1 ≤ k ≤ n, write the components of f on V as wk = uk + ivk. The real Jacobian of f is an m × n matrix 0 1 @uk @uk B @xj @yj C J f = B C R @ @vk @vk A @xj @yj which defines a linear map JRf : TpM ! Tf(p)N for any p 2 U. Extending scalars allows C C us to define the complex Jacobian JCf : Tp M ! Tf(p)N given by the same matrix JRf with n o n o respect to the real bases @ ; @ ; @ ; @ and @ ; @ ; @ ; @ of these tangent spaces. @xj @x¯j @yj @y¯j @uk @u¯k @vk @v¯k n o n o With respect to the complex bases @ ; @ and @ ; @ , the matrix is @zj @z¯j @wk @w¯k 0 1 @wk @wk B @zj @z¯j C J f = B C : C @ @w¯k @w¯k A @zj @z¯j Proposition 1.2.2. Let U ⊆ M; V ⊆ N and f : U ! V be as above. Then the following are equivalent: (1) f is holomorphic. (2) For all 1 ≤ j ≤ m and 1 ≤ k ≤ n, @wk = @w¯k = 0. @z¯j @zj 1;0 1;0 0;1 0;1 (3) For all p 2 U, JCf takes Tp M to Tf(p)N and Tp M to Tf(p)N. 4 1.2 Complex Manifolds 1 Complex Manifolds Corollary 1.2.3. Suppose M and N are complex manifolds of the same dimension and f : M ! N is holomorphic. Then 2 @wk det(JRf) = det(JCf) = det ≥ 0: @zj Corollary 1.2.4. A holomorphic map f : M ! N between complex manifolds of the same dimension is orientation-preserving. In particular, transition maps are orientation-preserving, so every complex manifold has a naturally defined orientation. Example 1.2.5. Note that the operators @ and @ act as expected on the coordinates @zj @z¯j 2 2 3 fzj; z¯jg. For example, consider the map f : C ! C, f(z; w) = z + w . Then JCf = 2z 3w which is surjective whenever (z; w) 6= (0; 0) so for any constant c 2 C r f0g, the equation z2 + w3 = c defines a smooth submanifold of C2. More generally, there are holomorphic versions of the inverse and implicit function theo- rems from differential geometry: Theorem 1.2.6 (Inverse Function Theorem). If f : M ! N is holomorphic and JCf is an isomorphism at p 2 M, then there is a neighborhood V ⊆ N of f(p) and an inverse f −1 : V ! f −1(V ) to f around p that is holomorphic on V . Theorem 1.2.7 (Implicit Function Theorem). Let f : M1 × M2 ! N be a holomorphic function such that JCf is invertible at (p; q) 2 M1 × M2 and set w = f(p; q). Then there is a unique holomorphic map g : M ! N such that f(p; g(p)) = w.
Details
-
File Typepdf
-
Upload Time-
-
Content LanguagesEnglish
-
Upload UserAnonymous/Not logged-in
-
File Pages32 Page
-
File Size-