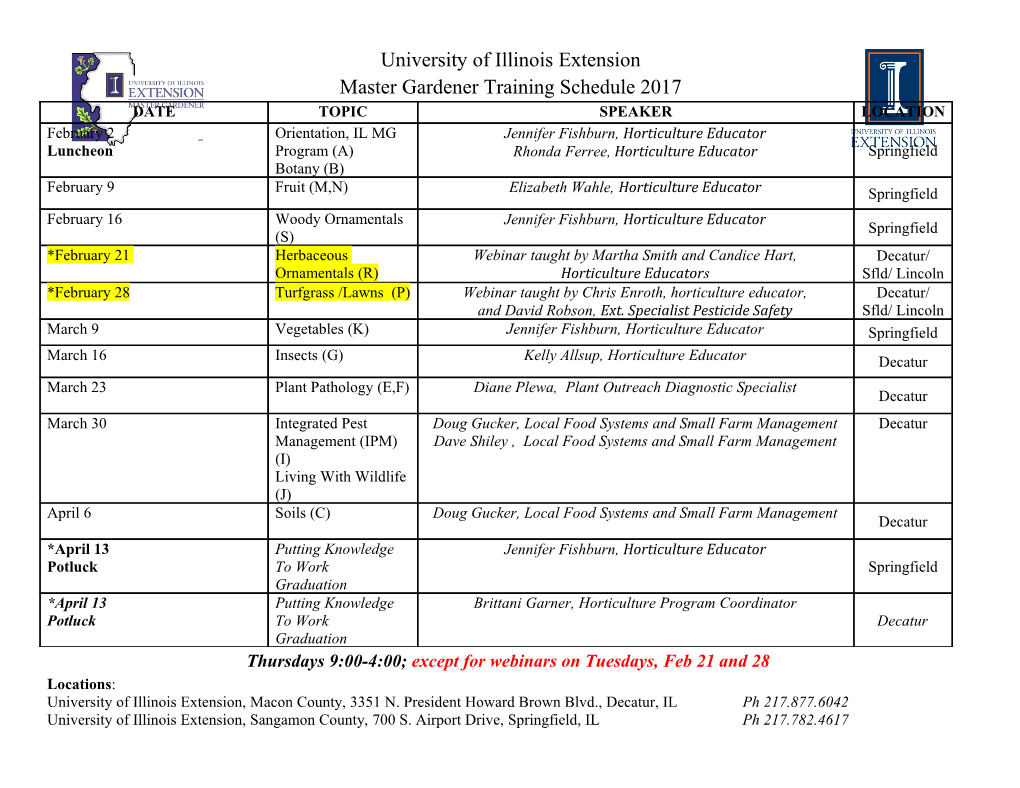
ANAEROBIC FERMENTATION OF WHEY: ACIDOGENESIS by WILLIAM SSEMPA KISAALITA B.Sc.(Eng.)(Hon.), Makerere Univesity, 1978 M.A.Sc., University of British Columbia, 1981 A THESIS SUBMITTED IN PARTIAL FULFILMENT OF THE REQUIREMENTS FOR THE DEGREE OF DOCTOR OF PHILOSOPHY in THE FACULTY OF GRADUATE STUDIES (Department of Chemical Engineering) We accept the thesis as conforming to the required standard THE UNIVERSITY OF BRITISH COLUMBIA January, 1987 © William Ssempa Kisaalita, 1987 In presenting this thesis in partial fulfilment of the requirements for an advanced degree at the University of British Columbia, I agree that the Library shall make it freely available for reference and study. I further agree that permission for extensive copying of this thesis for scholarly purposes may be granted by the head of my department or by his or her representatives. It is understood that copying or publication of this thesis for financial gain shall not be allowed without my written permission. Department of CtfBMlCfl-L- B-M G~( N &£rlQ-.| N (r- The University of British Columbia 1956 Main Mall Vancouver, Canada V6T 1Y3 Date <&( f /gT- ii ABSTRACT Based on the initial exploratory results of single-phase (acidogenesis and methanogenesis takes place in one vessel) whey biomethanation studies, a two-phase (acidogenesis and methanogenesis takes place in two separated serial vessels) biomethanation process was found to be more suitable for dealing with the current whey utilisation and/or disposal problem. Acidogenesis was found to be less understood in comparison to methanogenesis and therefore acidogenesis became the central problem of this thesis. Given that 90% of the five-day biochemical oxygen demand in whey is due to lactose, continuous culture (Chemostat) experiments were undertaken to examine the general mechanism of lactose acidogenesis by a mixed 14 undefined culture using C-labeled tracers. Also the influence of whey protein (mainly p-lactoglobulin) on the general fermentation scheme was addressed. Experimental factors included a pH range of 4.0 to 6.5, a mesophilic temperature of 35°C and a dilution rate (D) range of 0.05 to 0.65 h"1. At a fixed pH level, the observed variability in the main acidogenic end products (acetate, propionate, butyrate and lactate) with respect to D were found to be a consequence of the systematic separation of the various microbial groups involved in acidogenesis. Batch incubation of a 14 [ C(U)]-lactate tracer with chemostat effluent samples and preparative separation of the end products followed by a liquid scintillation assay of the location of the radio activity demonstrated that a microbial population shift with increasing D was responsible for disabling the conversion of iii lactate to other end products and hence the observed increase in lactate concentrations at high D values. 14 14 Further use of [ C(U)]-butyrate and [ C(2)]-propionate revealed the predominant carbon flow routes from pyruvate to the various end products. A qualitative lactose acidogenic fermentation model was proposed, in which lactose is converted to pyruvate via the Embden-Meyerhof-Parnas pathway. Pyruvate in a parallel reaction is then converted to lactate and butyrate. In the presence of hydrogen reducing methanogens lactate is converted to acetate in a very fast reaction and not propionate as previously believed-. The implications of these findings with regard to optimising the acidogenic phase reactor are discussed. Acidogenic fermentation of protein together with lactose did not affect the carbon flow scheme. In the D range of 0.05 to 0.15 h \ low pH (pH < 5.0) was found to favour the butyrate route at the expense of the lactate route and at high pH (pH > 5.5) the lactate route was favoured at the expense of the butyrate route, the pH region of 5.0 to 5.5 being the transition range. In order to describe the microbial growth, the Monod chemostat model was chosen among the various alternatives, because of its simplicity and its physico-chemical basis. The estimated model parameters are reported. iv TABLE OF CONTENTS Pages ABSTRACT ii TABLE OF CONTENTS iv LIST OF TABLES viii LIST OF FIGURES x NOMENCLATURE AND ABBREVIATIONS xiv ACKNOWLEDGEMENTS xvi I. INTRODUCTION 1 1.1 Whey 1 1.2 Whey Disposal and/or Utilization 4 1.3 Biomethanation Process 4 1.4 Whey Biomethanation 7 1.5 Two-Phase Process 11 1.6 Research Objectives 13 1.7 Scope of the Study 15 II. PREVIOUS WORK AND THEORETICAL ASPECTS 17 2.1 Energetics and Metabolic Stages in Anaerobiosis 17 2.1.1 Energetics 17 2.1.2 Metabolic Stages 19 2.1.2.1 Hydrolytic Bacteria 22 2.1.2.2 The ^-producing Bacteria 23 2.1.2.3 Homoacetogenic Bacteria 25 2.1.2.4 The Methanogenic Bacteria 25 2.1.2.5 Specific Bacterial Species Associated with Whey Biomethanation 27 2.1.2.6 Important Process Parameters 28 V Pages 2.2 Mathematical Modeling of Microbial Growth 28 2.2.1 Model Classification 28 2.2.2 Models for Single Substrate Limited Growth 31 2.2.2.1 Monod Equation 33 2.2.2.2 Other Equations 33 2.2.3 Models with Specific Growth Substrate and/or Biomass Growth Dependence 36 2.2.4 Microbial Growth Modeling Recommendation 39 2.2.5 Miscellaneous Models 39 2.2.5.1 Models of Growth in Presence of Inhibiting Substrate/Product 39 2.2.5.2 Models for Growth Limited by More Than One Substrate 41 2.2.5.3 Models for Product Formation 42 2.3 Mesophilic Microbial Kinetic Models: Literature Review .. 43 2.3.1 Whey and/or Lactose Substrates 43 2.3.2 Other Substrates 46 2.4 Mathematical Analysis of Continuous Culture 46 2.5 Experimental Plan 51 2.5.1 Assumptions 51 2.5.2 Experimental Factors 51 III. MATERIALS AND METHODS 53 3.1 Inoculum 53 3.2 Media 53 3.2.1 Lactose Limited Growth Sythetic Medium 53 3.2.2 Lactose/Protein Growth Medium 55 vi Pages 3.3 Fermentor Set-up 55 3.4 Fermentor Start-up and Operation Procedure 58 3.5 Set-up for Radioactive Tracer Incoporation 59 3.5.1 Apparatus with pH Control 59 3.5.2 Apparatus without pH Control 61 3.6 Preparative Separation of Organic Acids 61 3.6.1 Principle 61 3.6.2 Apparatus 64 3.6.3 Materials and Reagents 67 3.6.4 Procedure 67 3.7 Determination of Radioactivity 69 3.7.1 Principle 69 3.7.2 Channels Ratio Method 72 3.7.3 Procedure 72 IV. RESULTS AND DISCUSSION 75 4.1 Influence of Dilution Rate (D) on Organic Acids (OA) Distribution 76 4.2 Influence of D on Gaseous Products Distribution •••••••••• 82 4.3 Radio Tracer Studies 90 4.3.1 Results of the Radio Tracer Experiments at High D . 91 4.3.1 Results of the Radio Tracer Experiments at Low D .. 95 4.4 Proposed Lactose Acidogenic Fermentation Model 102 4.5 Significancy of Findings 108 4.6 Influence of Protein on the Fermentation Model Ill 4.7 Influence of pH on Carbon Flow from Pyruvate 115 4.8 Microbial Growth Model 117 vii Pages V. CONCLUSIONS AND RECOMMENDATIONS 132 5.1 Conclusions 132 5.2 Recommendations 135 LITERATURE CITED 136 APPENDIX A 155a APPENDIX B 173 APPENDIX C 183 APPENDIX D 188 viii LIST OF TABLES Pages Table 1.1 Typical Composition Whey Solids 2 Table 1.2 Estimated Quantities of Fluid Whey Produced in the USA 3 Table 1.3 Estimated Quantities of Fluid Whey Produced in Canada 3 Table 1.4 Two-Phase Pilot and Full Scale Plants 12 Table 2.1 Overall Reaction Schemes of Some Important Acidogenic Fermentation 20 Table 2.2 Generalised Growth Constants for Anaerobiosis 47 Table 2.3 Factors Investigated 52 Table 3.1 Lactose Limited Growth Medium 54 Table 3.2 Lactose and Protein Growth Medium Analysis 56 Table 4.1 Maximum Specific Growth Rate Values for Methanogenic Bacteria 89 Table 4.2 Radioactivity Distribution for High D Radio Tracer Experiments 94 Table 4.3 Radioactivity Distribution for Low D Radio Tracer Experiments 99 Table 4.4 Distribution of Total Free Energy Change for Growth of the Two-Phase Anaerobic Process of Glucose to Methane Over Different Microbial Groups 110 Table 4.5 Radioactive Distribution for Samples from Experiments With Lactose/Protein Substrate 116 ix Pages Table 4.6 Monod Chemostat Model Predictions for Different K s Values Based on u and Y Approximations for a pH of 6.0 129 Table 4.7 Comparisons of Monod Chemostat Model Constants for Acidogenesis 130 Table Bl Total Carbon Mass Balance (Lactose Growth Limited Substrate) at a pH of 6.0 and a Temperature of 35°C 174 Table B2 Total Carbon Mass Balance (Lactose/Protein Growth Limited Substrate) at a pH of 6.0 and a Temperature of 35°C 176 Table B3 Total Carbon Mass Balance (Lactose Growth Limited Substrate) at a pH of 4.5 and a Temperature of 35°C 177 Table B4 Total Carbon Mass Balance (Lactose Growth Limited Substrate) at a Dilution Rate of 0.05 h ^ and a Temperature of 35°C 178 Table B5 Total Gas Production as a Function of Cummulative Experimental Time at a Temperature of 25°C and a Pressure of One Atmosphere 179 X LIST OP FIGURES Pages Figure 1.1 Three Different Biomethanation Processes 6 Figure 1.2 Five Basic Anaerobic Fixed Film Reactor Types 8 Figure 1.3 Three Basic Anaerobic Flocs/Non-Attached Film Reactor Types 9 Figure 2.1 Overall Reaction Schemes of Some Important Acidogenic Fermentation Types 21 Figure 2.2 Effect of Partial Pressure of Hydrogen on the Free Energy Change for the Degradation of Ethanol, Propionate and Butyrate with Methane Formation from H2/C02 24 Figure 2.3 Possible Perspective Interactions for Cell Population Kinetic Representation 32 Figure 2.4 A Combination of Equations for the Substrate and/or Biomass Concentration Dependence of the Specific Growth Rate 40 Figure 2.5 The Ideal Continuous-Flow Stirred Tank Reactor (CSTR) ..
Details
-
File Typepdf
-
Upload Time-
-
Content LanguagesEnglish
-
Upload UserAnonymous/Not logged-in
-
File Pages208 Page
-
File Size-