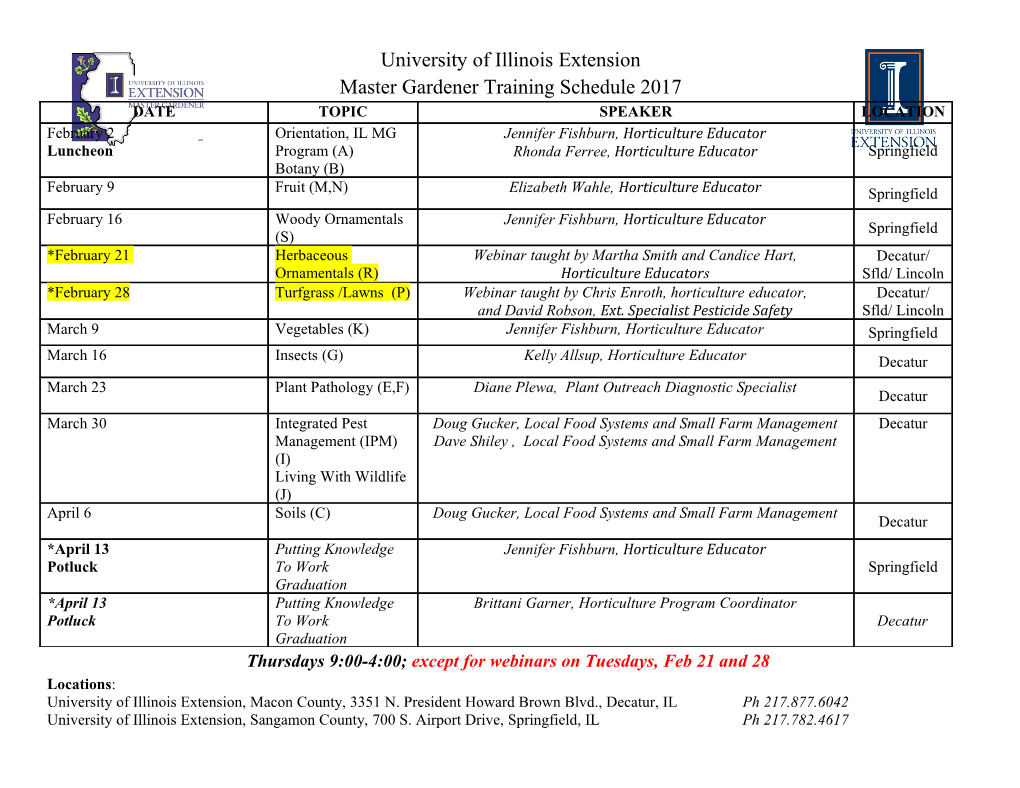
Thermopower in Two-Dimensional Electron Systems Thesis by William Elbridge Chickering In Partial Fulfillment of the Requirements for the Degree of Doctor of Philosophy California Institute of Technology Pasadena, California 2016 (Defended December 10, 2015) ii c 2016 William Elbridge Chickering All Rights Reserved iii Acknowledgements This thesis and the several years of research that preceded it could not have been com- pleted without the love and support of my colleagues, friends, and family. First and foremost, I want to thank my incredibly patient wife, Theresa, who was always there for me, vicariously enduring the highs and lows of my extended academic journey. I was fortunate to share my time at Caltech with many brilliant labmates. I am particularly indebted to Ghislain Granger who tutored me in the art of low temper- ature physics and device fabrication. Many thanks also go to Erik Henriksen and Johannes Pollanen who animated our underground laboratory with their good hu- mor and endless curiosity. And to Debaleena Nandi and Trupti Khaire for our many conversations about life and the world above ground. Finally, I must express profound gratitude to my advisor, Professor Jim Eisenstein. His passion, integrity, and generosity was not only instrumental for the work described in this thesis, but also provided me a model to aspire to both professionally and personally. iv Abstract The subject of this thesis is the measurement and interpretation of thermopower in high-mobility two-dimensional electron systems (2DESs). These 2DESs are realized within state-of-the-art GaAs/AlGaAs heterostructures that are cooled to tempera- tures as low as T = 20 mK. Much of this work takes place within strong magnetic fields where the single-particle density of states quantizes into discrete Landau levels (LLs), a regime best known for the quantum Hall effect (QHE). In addition, we review a novel hot-electron technique for measuring thermopower of 2DESs that dramatically reduces the influence of phonon drag. Early chapters concentrate on experimental materials and methods. A brief overview of GaAs/AlGaAs heterostructures and device fabrication is followed by de- tails of our cryogenic setup. Next, we provide a primer on thermopower that focuses on 2DESs at low temperatures. We then review our experimental devices, tempera- ture calibration methods, as well as measurement circuits and protocols. Latter chapters focus on the physics and thermopower results in the QHE regime. After reviewing the basic phenomena associated with the QHE, we discuss ther- mopower in this regime. Emphasis is given to the relationship between diffusion thermopower and entropy. Experimental results demonstrate this relationship per- sists well into the fractional quantum Hall (FQH) regime. Several experimental results are reviewed. Unprecedented observations of the diffusion thermopower of a high-mobility 2DES at temperatures as high as T = 2 K are achieved using our hot-electron technique. The composite fermion (CF) effective mass is extracted from measurements of thermopower at LL filling factor ν = 3=2. The thermopower versus magnetic field in the FQH regime is shown to be qualitatively v consistent with a simple entropic model of CFs. The thermopower at ν = 5=2 is shown to be quantitatively consistent with the presence of non-Abelian anyons. An abrupt collapse of thermopower is observed at the onset of the reentrant integer quantum Hall effect (RIQHE). And the thermopower at temperatures just above the RIQHE transition suggests the existence of an unconventional conducting phase. vi Contents Acknowledgements iii Abstract iv Introduction and Summary 1 1 Two-Dimensional Electron System 7 1.1 GaAs/AlGaAs Heterostructures . 9 1.2 Device Fabrication . 15 1.2.1 Hot-Electron Thermocouple . 19 1.2.2 2DES Diving Boards . 20 1.3 Summary . 22 2 Cryogenic Environment 24 2.1 Energy/Temperature Scales . 24 2.2 Dilution Refrigerators . 26 2.3 Cold Finger . 28 2.4 Connecting and Cooling the 2DES . 30 2.5 Sample Illumination . 32 2.6 \High" Temperature Regulation . 33 2.7 Summary . 33 3 Thermopower of 2D Electrons 35 3.1 The Seebeck Effect . 35 3.2 Classical Transport: Drude Model . 38 vii 3.3 Mott's Formula . 39 3.4 Thermopower vs Entropy . 42 3.5 Phonon Drag Thermopower . 44 3.6 Summary . 48 4 Diving Board Technique 49 4.1 Diving Board A . 50 4.1.1 Temperature Calibration . 51 4.1.2 Measurement Protocol . 56 4.1.3 Experimental Results at Zero Field . 59 4.1.4 Long Thermal Relaxation Times . 63 4.2 Diving Board B . 65 4.2.1 Short Thermal Relaxation Times . 67 4.2.2 Temperature Calibration . 68 4.2.3 Measurement Protocol . 70 4.3 Summary . 71 5 Hot-Electron Thermocouple 73 5.1 Device Layout . 74 5.2 Temperature Calibration . 77 5.3 Measurement Protocol . 81 5.4 Experimental Results . 82 5.5 Future Work: Metal-Insulator Transition . 88 5.6 Summary . 92 6 Quantum Hall Effect 94 6.1 Classical Hall Effect . 95 6.2 Landau Level Quantization . 98 6.3 Electron Spin . 102 6.4 Filling Factor . 103 6.5 Disorder Broadening . 104 viii 6.6 Compressibility . 106 6.7 Edge States . 108 6.8 Quantum Magnetotransport . 110 6.9 Quantum Lifetime . 115 6.10 Summary . 116 7 Thermopower in the Quantum Hall Regime 119 7.1 Classical 2DES in a Magnetic Field . 120 7.2 Thermoelectric Currents . 123 7.3 Thermopower with LL Quantization . 125 7.4 Entropic Model . 129 7.5 Disorder and the Generalized Mott Formula . 132 d d 7.6 Computing Sxx and Sxy . 133 7.7 Phonon Drag in the QH Regime . 136 7.8 Experimental Results at Low Fields . 138 7.9 Summary . 144 8 Fractional Quantum Hall Regime 146 8.1 Fractional Quantum Hall Effect . 147 8.1.1 Laughlin States . 148 8.1.2 Composite Fermions . 149 8.1.3 Wigner Crystal . 152 8.2 Thermopower in the FQH Regime . 153 8.2.1 CFs at B∗ =0........................... 154 8.2.2 Entropic Model . 158 8.2.3 Disorder . 160 8.3 Experimental Results at High Fields . 162 8.3.1 Sxx vs T at ν = 3=2 . 163 8.3.2 Comparison to Experiment on 2D Holes . 166 8.3.3 Comparison to Noninteracting Disordered Model . 168 8.3.4 Sxx vs B in FQH Regime . 168 ix 8.3.5 Low-Temperature Fluctuations . 174 8.3.6 Comparison to Entropic Model . 176 8.4 Summary . 179 9 The First Excited Landau Level 181 9.1 FQH State at ν = 5=2 . 182 9.1.1 Non-Abelian Anyons . 184 9.1.2 Thermopower of Non-Abelian Anyons . 186 9.1.3 Experimental Results . 188 9.2 Reentrant Integer Quantum Hall Effect . 192 9.2.1 N ≥ 2 LLs . 192 9.2.2 N = 1 LL . 194 9.2.3 Experimental Results . 197 9.3 Summary . 200 A Properties of a 2DES in GaAs 202 B Sample Processing 203 B.1 Sample Cleaving . 203 B.2 Cleaning the Sample . 204 B.3 Photolithography . 204 B.4 Etching the Mesa . 206 B.5 Ohmic Contacts . 206 B.5.1 InSn Ohmic Contacts . 207 B.5.2 Ni/AuGe Ohmic Contacts . 208 B.6 Al Top Gates . 209 B.7 Thin Sample . 209 B.8 Mount Diving Board . 211 B.9 Attach Heater . 213 B.10 Wire Up . 214 x C Narrow Band Lock-In Detection 216 C.1 Example: Four-Point Resistance Measurement . 216 D Long Thermal Relaxation Times 218 E Electrical Resistance as a Hot-Electron Thermometer 221 E.1 Introduction . 221 E.2 Error in ∆R Due to Joule Heating . 222 E.3 Error in ∆T Due to Joule Heating . 223 E.4 Impact on Thermopower Measurement . 224 F Corbino Thermopower 225 F.1 Corbino vs Hall Bar . 229 G Diffusion Thermopower of a Band Insulator 232 Bibliography 236 xi List of Figures 1.1 Band structure of a AlGaAs-GaAs heterojunction . 10 1.2 Illustration of AlGaAs-GaAs-AlGaAs quantum well . 11 1.3 MBE grown epilayers of a quantum well heterostructure . 12 1.4 Conduction band of delta-doped GaAs/AlGaAs quantum well . 14 1.5 Simple 2DES device. 15 1.6 Lithographically patterned 2DES device..
Details
-
File Typepdf
-
Upload Time-
-
Content LanguagesEnglish
-
Upload UserAnonymous/Not logged-in
-
File Pages260 Page
-
File Size-