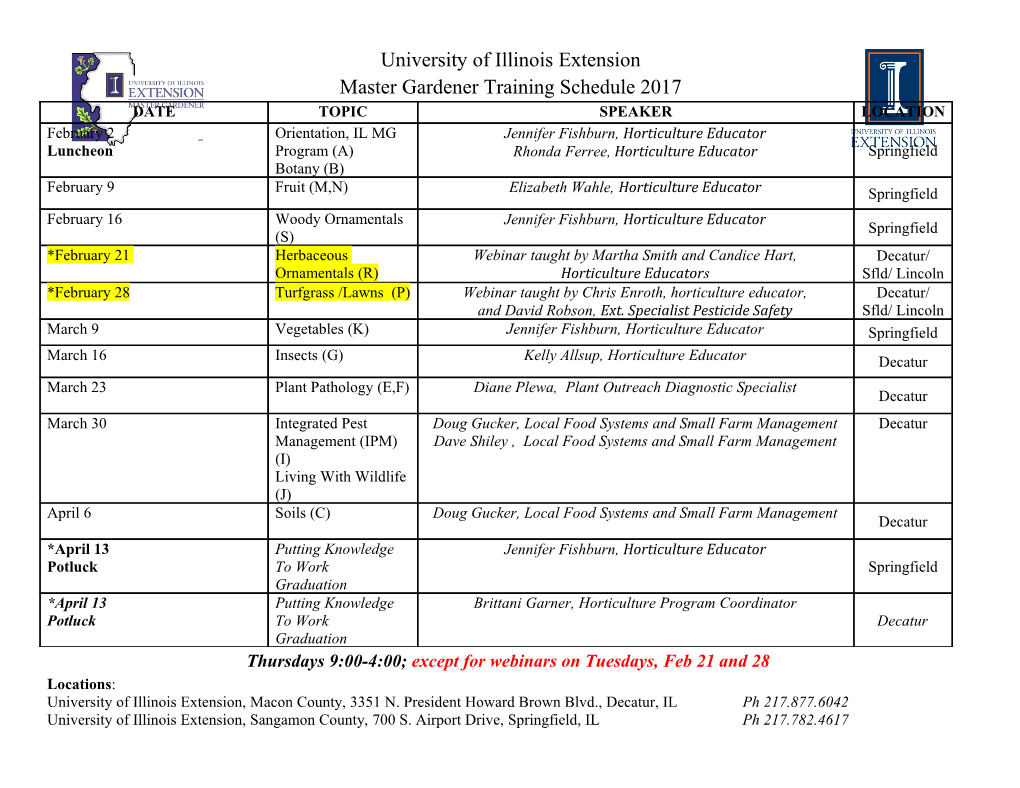
Desalination and Water Treatment 170 (2019) 415–424 www.deswater.com December doi: 10.5004/dwt.2019.24837 Evaluation of fouling prevention methods in a submerged membrane bioreactor treating domestic wastewater Konstantinos Azis, Spyridon Ntougias, Paraschos Melidis* Laboratory of Wastewater Management and Treatment Technologies, Department of Environmental Engineering, Democritus University of Thrace, Vas. Sofias 12, Xanthi 67132, Greece, Tel. +30-25410-79372; emails: [email protected] (P. Melidis), [email protected] (K. Azis), [email protected] (S. Ntougias) Received 9 April 2019; Accepted 27 August 2019 abstract Membrane fouling is considered as the main drawback that currently restricts use of membrane bioreactors (MBR) in domestic wastewater treatment. Microbiological factors such as extracellular polymeric substances (EPS) and soluble microbial products (SMP) have a critical impact on sludge filterability of membrane surfaces. The effects of operating parameters and sludge characteristics on fouling were examined in a pilot-scale MBR treating domestic wastewater under alternating aeration and intermittent feeding conditions. EPS and SMP were measured under various operat- ing conditions and their effects on membrane fouling were assessed. Both glycerol and biosolids addition resulted in carbohydrate EPS and SMP increase, whereas operating mixed liquor tempera- ture above 19°C drastically reduced membrane flux as the consequence of the enhanced microbial activity. The first transmembrane pressure derivative (dTMP/dt) proved to be a suitable indicator of critical flux since an acute raise in dTMP/dt occurred. The implementation of various fouling prevention methods, such as increased backwash flow rate, cross flow aeration, in situ mechanical cleaning, anoxic/aerobic phase duration ratio and offline intensive chemical cleaning, resulted in the improvement of membrane permeability (P). Moreover, the increase in anoxic/aerobic phase ratio accelerated membrane fouling. Keywords: Extracellular polymeric substances (EPS); Soluble microbial products (SMP); Membrane resistance (R); TMP first derivative; Anoxic/aerobic cycle; Wastewater treatment 1. Introduction highly affect membrane fouling [3–5]. Temperature is a bio- kinetic factor, has a direct impact on biosolids generation [3] Fouling in membrane treating wastewater increases and subsequently on membrane fouling [6]. Nonetheless, energy requirement and minimizes membrane lifespan, the temperature is not the only parameter that influences resul ting in higher operating costs [1]. Microbial aggregates membrane resistance to filtration [6–8]. Sludge character- deposited on membrane pores increase membrane resis- istics and in particular extracellular polymeric substances tance, creating a more intense and irreversible membrane (EPS) and soluble microbial products (SMP) concentrations clogging [2]. The key factors that affect the permeability affect membrane resistance [1,9], increasing membrane are the membrane material, the mixed liquor characteristics, cleaning frequency. A range of factors, such as composition the strength and the composition of the influent. Moreover, of wastewater, SRT, mixed liquor suspended solids (MLSS) the main operating parameters, such as the sludge retention and bacterial growth rate, have strong impact on EPS and time (SRT) and food-to-microorganism ratio (F/M ratio), SMP levels [5,10]. * Corresponding author. Presented at the Fifth International Conference on Small and Decentralized Water and Wastewater Treatment Plants (SWAT 2018), 26–29 August 2018, Thessaloniki, Greece 1944-3994/1944-3986 © 2019 Desalination Publications. All rights reserved. 416 K. Azis et al. / Desalination and Water Treatment 170 (2019) 415–424 Fouling control can be achieved by altering the operat- period, sludge was not wasted out and the corresponding ing conditions and optimizing bioprocesses as well as by sludge age SRT was identical with the exact operating day. applying physico-chemical cleaning methods. An exten- The external tank was equipped with three flat-sheet sive num ber of techniques has been implemented to restrict ultrafiltration membranes (Microdyn Nadir UP-150, flux decline [5,11]. Emerging fouling prevention methods Wiesbaden, Germany). More details about the membrane includes the regulation of the air flow velocity [12], the technical specifications can be found in Azis et al. [29]. Fine increase in backwash flow rate [13–15], the implementation bubble aeration diffuser was located in the membrane tank to of intermittent aeration strategies [16–18] and the perfor- provide air crossflow through an air-blower, thus preventing mance of the filtration process below the estimated critical membrane clogging [30]. The level of dissolved oxygen was flux [19]. Intermittent aeration is a well-established method to maintained within 2 and 3.5 mg L–1 in both tanks during the control membrane fouling with reduced energy requirement aerobic phase. Internal mixing of the activated sludge inside [12,16,19]. Mechanical scouring practices can decrease energy the membrane tank was achieved by a submersible pump consumption in aerobic membrane bioreactor (MBRs). On the operating at 2,500 L h–1. 3 –1 other hand, mechanical fouling mitigation approaches are The input flow rate (Qin) was 0.18 m d , corresponding to limited, therefore in this study, pneumatic cleaning of the a hydraulic retention time of 1 d. MBR flow rate (Qef) ranged membrane by air sparging was investigated. Membrane between 0.048 and 0.18 m3 d–1, depending on the membrane cleaning through utilization of the mechanical properties fouling events. The temperature of the mixed liquor was of a sponge is an alternative way to control fouling. [20,21]. fluctuated from 15°C to 22°C. The TMP was monitored by a Moreover, in situ and ex situ mechanical cleaning practices transducer and the obtained data were logged per min via can improve cleaning efficacy [22]. the Modscan32 software. The permeate and back- flushing Chemical cleaning is implemented in emergency fouling duration were 8 and 1 min, respectively, with intermediate incidents, which are known as irreversible fouling, where relaxation periods of 30 s (total filtration cycle, 10 min). In strongly absorbing deposits are removed [23]. Organic foul- order to control the membrane fouling, the pilot-plant was ing is due to proteins, polysaccharides, humic acids and other operated below the critical flux. Critical flux was estimated organic molecules [24]. However, the frequency of chemical by the flux-step method [27]. cleaning can be minimized by applying the abovementioned Measurement of membrane resistance and permeability physical methods. An alternative approach to prevent mem- was based on Darcy’s law (Eqs. 1–3): brane fouling on long-term basis is bioreactor operation under the critical flux [25], which is commonly determined TMP by the flux-step method [25–27]. In particular, some stud- R = (1) Jn× ies have suggested that the critical flux in MBR systems should be set at the two-third of the maximum flux to sustain effective membrane operation, where both transmembrane J P = (2) pressure (TMP) and flux profile are linearly correlated [28]. TMP The scope of this study was to prevent membrane fouling in MBRs for extended time period by controlling critical flux −−11� and consequently the effects of EPS and SMP on membrane RP=×n (3) permeability under various operating conditions, including temperature and anoxic/aerobic cycle ratio. Moreover, the where R is the membrane resistance (m–1); P is the permea- appropriate fouling prevention methods should be followed bility (L m–2 h–1 bar–1); TMP is the transmembrane pressure are proposed in relationship to membrane resistance. (mbar or bar, as appropriate); J is the permeate flux (L m–2 h–1); and n is the activated sludge viscosity (kg m–1 s–2). 2. Materials and methods 2.1. Experimental setup and operating conditions 2.2. Cleaning methods A pilot-scale MBR system that consists of a 40 L of feeding Mechanical cleaning was applied either by water pres- tank, a 100 L of bioreactor and an 80 L of external membrane sure or by the aid of a sponge with thin folds, remov- tank was installed (Fig. 1). A BE/M-25 CE ROVER brass pump ing membrane surface particles. Taking advantage of the was placed within the two tanks, resulting in the recircula- sponge geometry and the sponge material characteristics, tion of the activated sludge from the external MBR tank back successful removal of the build-up cake layer from mem- to the cylindrical bioreactor by operating 20 s every 3 min at brane sheets occurred. The alternating aeration approach 28 L min–1, corresponding to a mean flow rate of 187 L h–1. was applied under the following anoxic/aerobic phase dura- A radial piston stirrer was setup in the main bioreactor and a tion ratios (min/min): 30/60, 60/60 and 90/60. As an addi- blower supplied air into a fine bubble diffuser plate at a rate of tional fouling prevention method, the backwash flow rate 1.5 L m–1. The start-up of MBR system was achieved through was increased from 200 to 400 mL min–1 and the air flow the addition of mixed liquor from the wastewater treatment rate was elevated from 6 to 8 L min–1. The membrane mod- plant of Xanthi, Greece. The cycling feeding at the beginning ule was subjected to ex situ intensive chemical cleaning by of the anoxic phase enhanced the simultaneous removal of applying 500–1,000 mg L–1 NaOCl solution for a period of the nutrients (C, N and P) [29]. The MBR was operated under 24 h. The membrane tank remained inactive during this an organic loading rate of 0.27 ± 0.02 g biochemical oxygen cleaning period, where biosolids were transferred to the –1 –1 demand (BOD5) g VSS d . During the entire experimental main bioreactor. K. Azis et al. / Desalination and Water Treatment 170 (2019) 415–424 417 1– Peristaltic pump ║ 9– Air-flow meter 2– Overhead stirrer ║ 10– Air blower 3– Flow divider ║ 11– Digital pressure transmitter 4– Equalization tank ║ 12– Pressure gauge 5– Air diffuser plate ║ 13– PC ║ 14– Flat sheet UF 6– Radial piston stirrer ║ 15– Submersible pump 7– Bioreactor tank ║ 16– Membrane tank 8– Recirculation pump Fig.
Details
-
File Typepdf
-
Upload Time-
-
Content LanguagesEnglish
-
Upload UserAnonymous/Not logged-in
-
File Pages10 Page
-
File Size-