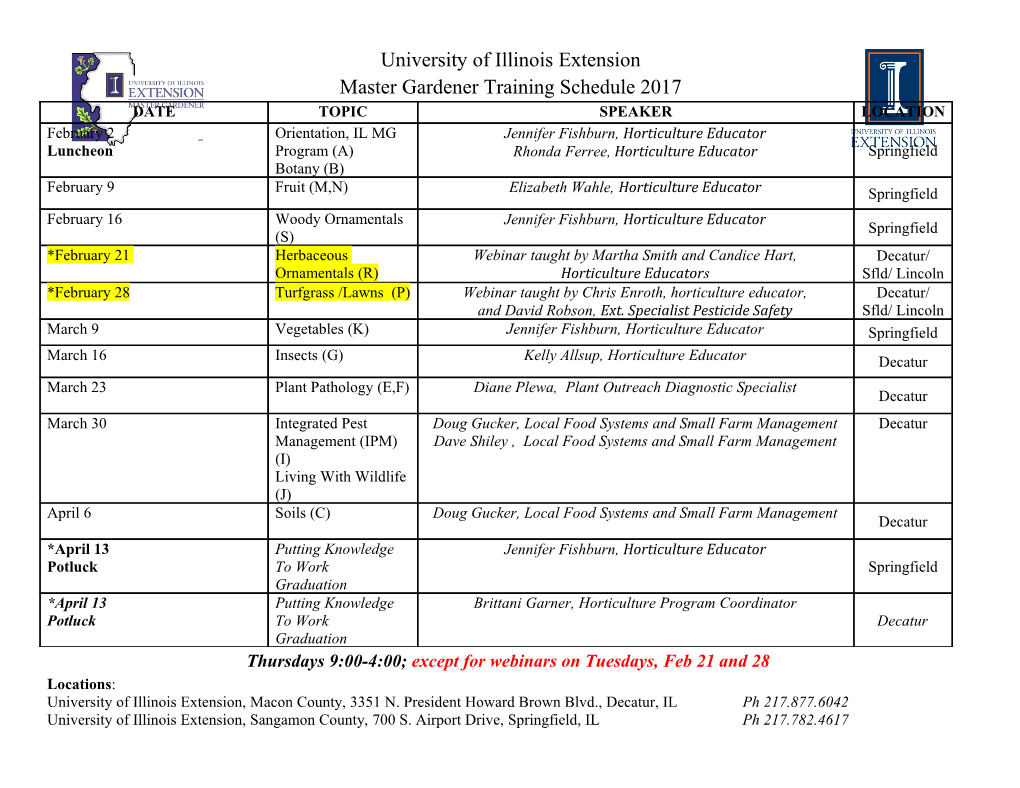
bioRxiv preprint doi: https://doi.org/10.1101/2020.02.10.941245; this version posted September 10, 2020. The copyright holder for this preprint (which was not certified by peer review) is the author/funder, who has granted bioRxiv a license to display the preprint in perpetuity. It is made available under aCC-BY 4.0 International license. 1 Association of gut microbiota with metabolism in juvenile Atlantic Salmon 2 Dvergedal, H.1, Sandve, S.R.1*, Angell, I.L.2, Klemetsdal, G.1, Rudi, K.2 3 4 1Department of Animal and Aquacultural Sciences, Faculty of Biosciences, Norwegian University 5 of Life Sciences, P. O. Box 5003, NO-1433, Aas, Norway 6 2Faculty of Chemistry, Biotechnology and Food Science, Norwegian University of Life Sciences, 7 P. O. Box 5003, NO-1433, Aas, Norway 8 9 *Correspondence: Simen Rød Sandve, Norwegian University of Life Sciences, NO-1433 Aas, 10 Norway. [email protected] bioRxiv preprint doi: https://doi.org/10.1101/2020.02.10.941245; this version posted September 10, 2020. The copyright holder for this preprint (which was not certified by peer review) is the author/funder, who has granted bioRxiv a license to display the preprint in perpetuity. It is made available under aCC-BY 4.0 International license. 11 Abstract 12 The gut microbiome plays a key role in animal health and metabolism through the intricate 13 functional interconnection between the feed, gut microbes, and the host. Unfortunately, in 14 aquaculture, the links between gut microbes and fish genetics and production phenotypes are not 15 well understood. 16 In this study, we investigate the associations between gut microbial communities, fish feed 17 conversion, and fish genetics in the domestic Atlantic salmon. Microbial community composition 18 was determined for 230 juvenile fish from 23 full-sib families and was then regressed on growth, 19 carbon and nitrogen metabolism, and feed efficiency. We only found weak associations between 20 host genetics and microbial composition. However, we did identify significant (p < 0.05) 21 associations between the abundance of three microbial operational taxonomical units (OTUs) and 22 fish metabolism phenotypes. Two OTUs were associated with both carbon metabolism in adipose 23 tissue and feed efficiency, while a third OTU was associated with weight gain. 24 In conclusion, this study demonstrates an intriguing association between host lipid metabolism and 25 the gut microbiota composition in Atlantic salmon. 26 27 Keywords: microbiome, Atlantic salmon, genetics, metabolism, feed efficiency, carbon turnover 28 29 30 bioRxiv preprint doi: https://doi.org/10.1101/2020.02.10.941245; this version posted September 10, 2020. The copyright holder for this preprint (which was not certified by peer review) is the author/funder, who has granted bioRxiv a license to display the preprint in perpetuity. It is made available under aCC-BY 4.0 International license. 31 Background 32 Efficient and environmentally sustainable animal production systems are urgently required to 33 ensure long-term food security, especially as global aquaculture consumption is projected to 34 double by 2050 (www.fao.org). One important aspect of improving sustainability is to improve 35 feed conversion and growth. In humans and other vertebrate systems, the gut microbiome plays a 36 central role in the path from “feed-to-animal” (1–4), and recent studies have also shown that host- 37 genetic factors can modulate microbiome composition. Such functional interconnection between 38 feed, microbes, and host (i.e. the feed-microbiome-host axis), opens up intriguing avenues for 39 optimizing aquaculture production systems, for example by breeding for ‘optimized’ microbiome 40 composition (5). 41 Yet, even though the dietary composition is known to impact the gut microbiome in aquaculture 42 species (1), almost nothing is known about the link between the gut microbiota and important 43 production phenotypes, or to what extent microbiota composition itself could be a new breeding 44 target for aquaculture breeding programs (5). 45 To address this pressing knowledge gap we use a family-based experimental design to test if 46 variation in the gut microbiome composition in juvenile Atlantic salmon is associated with key 47 phenotypes related to host metabolism as well as variation in host genetics. Our results identified 48 phenotypic associations between host gut microbiome and lipid metabolism, growth, as well as to 49 feed efficiency, which open the possibility for metabolic modulation through the gut microbiota. 50 51 52 53 bioRxiv preprint doi: https://doi.org/10.1101/2020.02.10.941245; this version posted September 10, 2020. The copyright holder for this preprint (which was not certified by peer review) is the author/funder, who has granted bioRxiv a license to display the preprint in perpetuity. It is made available under aCC-BY 4.0 International license. 54 Materials and methods 55 Experimental setup 56 A family experiment with Atlantic salmon was carried out at the fish laboratory, Norwegian 57 University of Life Sciences (NMBU), Aas, Norway, according to the laws and regulations 58 controlling experiments on live animals in EU (Directive 2010/637EU) and Norway (FOR-2015- 59 06-18-761). The experiment was approved by the Norwegian Food Safety Authority (FOTS ID 60 11676). 61 The family experiment is explained in detail by Dvergedal et al (6). In short, broodstock from 62 AquaGen’s breeding population (22 males and 23 females) were used to generate 23 families. To 63 ensure clearly contrasted family groups with respect to growth potential, the parents were selected 64 in two directions for high and low estimated breeding values (EBVs) for growth in seawater, 65 respectively. 66 Prior to the start-feeding several families were kept in separate compartments within the same 67 tank, and five tanks were needed to house all families. Based on parentage assignment 100 family 68 members were identified for each of the 23 families and reared together in a single tank from start- 69 feeding until the start of the experiment. A priori to the 12-day test, families were allocated to 70 tanks, 50 fish per tank and 2 tanks per family (except for nine tanks in which the number of fish 71 varied between 42 and 54, due to some mortality prior to the start of the experiment or an increased 72 number due to a counting mistake). From each tank five fish (10 fish per family) were collected 73 for microbiota and phenotypic analyses, a total of 230 fish were sampled all together (Fig. 1). 74 Families were fed a fishmeal-based diet labeled with the stable isotopes 15N and 13C, with inclusion 75 levels of 2% and 1%, respectively, as described in Dvergedal et al. (6). bioRxiv preprint doi: https://doi.org/10.1101/2020.02.10.941245; this version posted September 10, 2020. The copyright holder for this preprint (which was not certified by peer review) is the author/funder, who has granted bioRxiv a license to display the preprint in perpetuity. It is made available under aCC-BY 4.0 International license. 76 The tanks, each with a 270-L capacity, were supplied with water from a common source of 77 recirculated fresh water, at a flow rate of 7 to 8 L.min-1. The fish were kept under 24 h light regime, 78 with an average temperature of 14.5°C. Dissolved oxygen was measured daily and maintained 79 above 8 mg.L-1 in the outlet water (Handy Delta, OxyGuard® AS, Farum, Denmark). 80 81 82 Figure 1. Schematic outline of the experimental setup. Twenty-three families were distributed on 46 tanks (two 83 tanks per family). Dominant live bacteria were identified prior to association analyses to fish genetics and metabolism. 84 85 Microbiota analyses 86 Distal intestinal samples (n = 230) were obtained by squeezing out the gut segment content using 87 sterile tweezers in 1 ml phosphate buffered saline (PBS) and put on ice until further processing. 88 To distinguish between DNA from dead and alive bacteria, the samples were treated with 89 propidium monoazide (PMA) within eight hours post sampling in order to inactivate free DNA, 90 and DNA in dead cells (7). Samples were pulse centrifuged up to 1200 rpm and split in two, where 91 one part (n = 230, PMA treated samples) was added PMA dye (Biotium, USA to a final 92 concentration of 50 µM, and the other part was kept as a control (n = 230, non-PMA treated 93 samples) with no added PMA. The samples were then kept dark for 5 min before exposure to light bioRxiv preprint doi: https://doi.org/10.1101/2020.02.10.941245; this version posted September 10, 2020. The copyright holder for this preprint (which was not certified by peer review) is the author/funder, who has granted bioRxiv a license to display the preprint in perpetuity. It is made available under aCC-BY 4.0 International license. 94 for 30 min in a lightbox from Geniul. DNA extraction (n = 460) was done using mag midi DNA 95 extraction kit (LGC Genomics, UK) following the manufacturer's recommendations. 96 The 16S rRNA amplicon library was prepared and sequenced as previously described (8). Briefly, 97 this involved amplification in 25-µl volumes, with 0.2 µM of both primers, and 2 µl genomic 98 DNA. The PCR cycles involved denaturation at 95°C for 30 s, annealing at 55°C for 30 s, with an 99 initial heat activation at 95°C for 15 min. Illumina modified adapters added with 10 new PCR 100 cyles after purification with AMPure XP beads (Beckman-Coulter, USA) were. Negative controls 101 without genomic DNA were included on all PCR plates (n=5), and included in sequencing if giving 102 detectable band by agarose gel electrophoresis.
Details
-
File Typepdf
-
Upload Time-
-
Content LanguagesEnglish
-
Upload UserAnonymous/Not logged-in
-
File Pages25 Page
-
File Size-