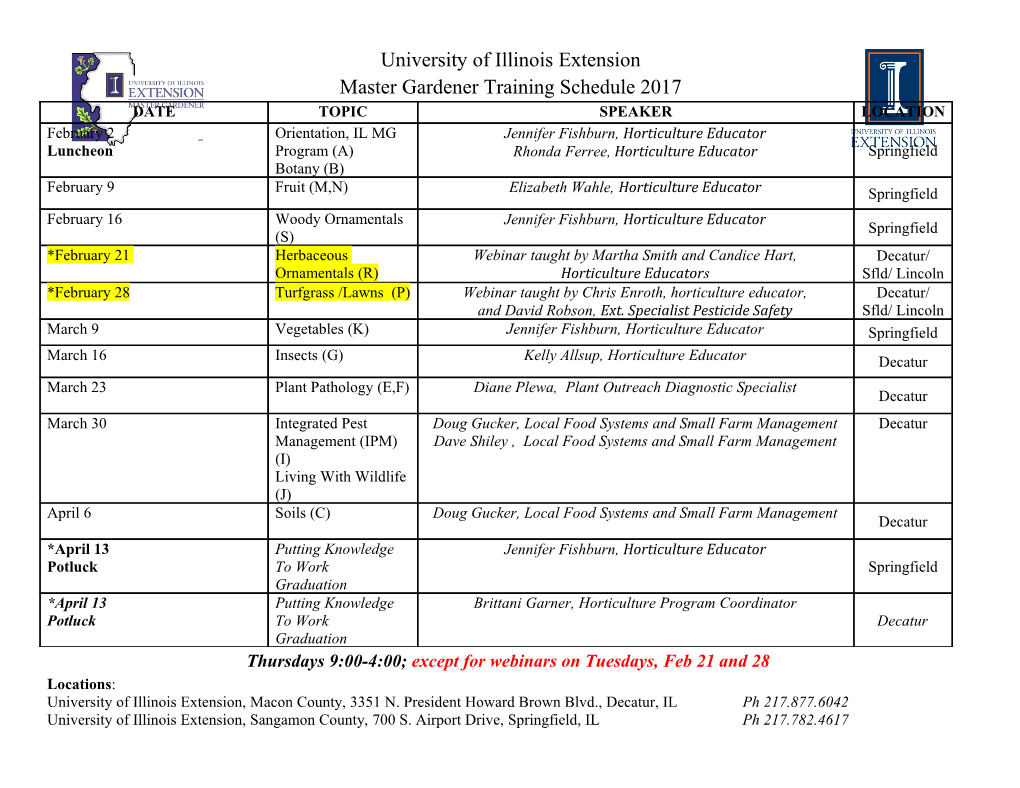
A NON SUPERREACTIVE ANTENNAS MAXIMUM DIRECTIVITY BOUNDING AND ITS RADIATION APERTURE MD. Javeed Ahammed1,Dr. R.P. Singh2 1(Research Scholar, Sri Satya Sai University of Technology and Medical Sciences, India) 2(Guide, Sri Satya Sai University of Technology and Medical Sciences, India) ABSTRACT Among the earliest type of antennas in production were aperture type. These antennas were rigid and consisted of a parabolic, paraboloidal, cylindrical, or spherical shape. A major limitation of this type of antenna stems from the fact they could only give one particular radiation pattern, and if one wanted to scan the signal from one point to another, then the whole structure had to be moved which meant the satellite had to be realigned. This major shortcoming led to the development of the more costly phased array technology and other technologies where beam scanning was exploited. The aperture is defined as the area, oriented perpendicular to the direction of an incoming radio wave, which would intercept the same amount of power from that wave as is produced by the antenna receiving it. At any point, a beam of radio waves has an irradiance or power flux density (PFD) which is the amount of radio power passing through a unit area of one square meter. I.INTRODUCTION With the advent of adaptive materials, active antennas are now being developed that employ piezoelectric stacks or electrostatic actuation mainly for optical and far field pattern correction [2,3]. The antennas proposed in this study will be utilized for beam scanning and shaping purposes where larger deflections are needed. Unlike the aperture antennas of old, these antennas are lightweight, flexible and can be designed to produce a "customer defined" far field radiation pattern. Recent theoretical and experimental studies have shown that by varying the surface of a reflector one can produce antennas that can achieve "customer defined" patterns while simultaneously adding the advantage of shape variation [4,5]. Reflector aperture variation achieves most of the objectives of phased array technology while adding the ability to correct for surface distortions caused by weather, thermal gradients, and atmospheric effects. An added feature of these antennas, which is of great military benefit, is the ability to transmit to a large area for maximum ground coverage and then by varying the antenna shape transmit to a very small area for security reasons.Recent studies have shown that reflector surface adaptation can achieve performance characteristics on the order of phase array antennas without the complexity 1171 | P a g e and cost. The work proposed in this study develops a class of antennas capable of variable directivity (beam steering) and power density (beam shaping). The actuation for these antennas is employed by either polyvinylidene fluoride (PVDF) film bonded to a metalized mylar skin or shape memory alloys (SMA) embedded in a composite structure. Theoretical studies of flexible antennas have shown that cylindrical antennas can achieve a directivity variation (beam scanning) of over 10° and an increase in ground coverage of over 40%. II.APERTURE EFFICIENCY In general, the aperture of an antenna is not directly related to its physical size. However some types of antennas, for example parabolic dishes and horns, have a physical aperture (opening) which collects the radio waves. In these aperture antennas, the effective aperture Aeff must always be less than the area of the antenna's physical aperture Aphys, as can be seen from the definition above. An antenna's aperture efficiency, ea is defined as the ratio of these two areas: ea=Aeff/Aphys The aperture efficiency is a dimensionless parameter between 0 and 1.0 that measures how close the antenna comes to using all the radio power entering its physical aperture. If the antenna were perfectly efficient, all the radio power falling within its physical aperture would be converted to electrical power delivered to the load attached to its output terminals, so these two areas would be equal Aeff = Aphys and the aperture efficiency would be 1.0. But all antennas have losses, such as power dissipated as heat in the resistance of its elements, non- uniform illumination by its feed, and radio waves scattered by structural supports and diffraction at the aperture edge, which reduce the power output. Aperture efficiencies of typical antennas vary from 0.35 to 0.70 but can range up to 0.90. III.APERATURE AND GAIN The directivity of an antenna, its ability to direct radio waves in one direction or receive from a single direction, is measured by a parameter called its gain, which is the ratio of the power received by the antenna to the power that would be received by a hypothetical isotropic antenna, which receives power equally well from all directions. 2 Aeff = /4 where λ is the wavelength of the radio waves. So the gain of any antenna is proportional to its aperture: 1172 | P a g e So antennas with large effective apertures are high gain antennas, which have small angular beam widths. Most of their power is radiated in a narrow beam in one direction, and little in other directions. As receiving antennas, they are most sensitive to radio waves coming from one direction, and are much less sensitive to waves coming from other directions. Although these terms can be used as a function of direction, when no direction is specified, the gain and aperture are understood to refer to the antenna's axis of maximum gain, or boresight. IV.RADIATION PATTERN Radiation pattern is one of the important characteristic of an antenna as tells the spatial relative distribution of the electromagnetic wave generated by the antenna. The radiation pattern is a plot of the magnitude of the radiation field as a function of direction (θ,ɳ ). The radiation pattern is essentially a 3-D surface. In the field of antenna design the term radiation pattern (or antenna pattern or far-field pattern) refers to the directional (angular) dependence of the strength of the radio waves from the antenna or other source. Particularly in the fields of fiber optics, lasers, and integrated optics, the term radiation pattern, or near-field radiation pattern, may also be used as a synonym for the near-field pattern or Fresnel pattern. This refers to the positional dependence of the electromagnetic field in the near-field, or Fresnel region of the source. The near-field pattern is most commonly defined over a plane placed in front of the source, or over a cylindrical or spherical surface enclosing it. The far-field pattern of an antenna may be determined experimentally at an antenna range, or alternatively, the near-field pattern may be found using a near-field scanner, and the radiation pattern deduced from it by computation. The far-field radiation pattern can also be calculated from the antenna shape by computer programs such as NEC. Other software, like HFSS can also compute the near field. The far field radiation pattern may be represented graphically as a plot of one of a number of related variables, including; the field strength at a constant (large) radius (an amplitude pattern or field pattern), the power per unit solid angle (power pattern) and the directive gain. Very often, only the relative amplitude is plotted, normalized either to the amplitude on the antenna boresight, or to the total radiated power. The plotted quantity may be shown on a linear scale, or in dB. The plot is typically represented as a three dimensional graph (as at right), or as separate graphs in the vertical plane and horizontal plane. This is often known as a polar diagram. Fig 1 Radiation Pattern 1173 | P a g e There are various parts of radiation pattern: 1. HPBW: The half power beamwidth (HPBW) can be defined as the angle subtended by the half power points of the main lobe. 2. Main Lobe: This is the radiation lobe containing the direction of maximum radiation. 3. Minor Lobe: All the lobes other then the main lobe are called the minor lobes. These lobes represent the radiation in undesired directions. The level of minor lobes is usually expressed as a ratio of the power density in the lobe in question to that of the major lobe. This ratio is called as the side lobe level (expressed in decibels). 4. Back Lobe: This is the minor lobe diametrically opposite the main lobe. 5. Side Lobes: These are the minor lobes adjacent to the main lobe and are separated by various nulls. Side lobes are generally the largest among the minor lobes. V.EFFECT ON ANTENNA LOSSES To obtain quantitative results for the effect of conduction losses on antenna performance an idealized model is again postulated. Intuitively one 'would expect the losses on a metal antenna to be smaller the more effectively the sphere is utilized. Therefore, for the ideal lossy antenna is postulated a spherical conductor of radius R excited by the magnetic sourceson its surface. So long as the sphere is a good conductor the source will generate the desired field [4]. If the conductivity of the sphere is poor, can be modified to allow for a field internal to the conductor. VI.CONCLUSION An optimized aperture distribution is investigated to achieve the maximum endfire directivity for a continuous line source with a uniform phase progression. The optimization method has the advantages of simplicity and efficiency, in which the aperture distribution is expanded into a set of cosine functions and the optimization reduces to solving a constrained linear-least-squares problem. Based on the copious computed results from the optimization, an approximate closed-form expression is derived for the maximum directivity. The expression can serve as a bound for the endfire directivity for a line source with a uniform phase progression.
Details
-
File Typepdf
-
Upload Time-
-
Content LanguagesEnglish
-
Upload UserAnonymous/Not logged-in
-
File Pages6 Page
-
File Size-