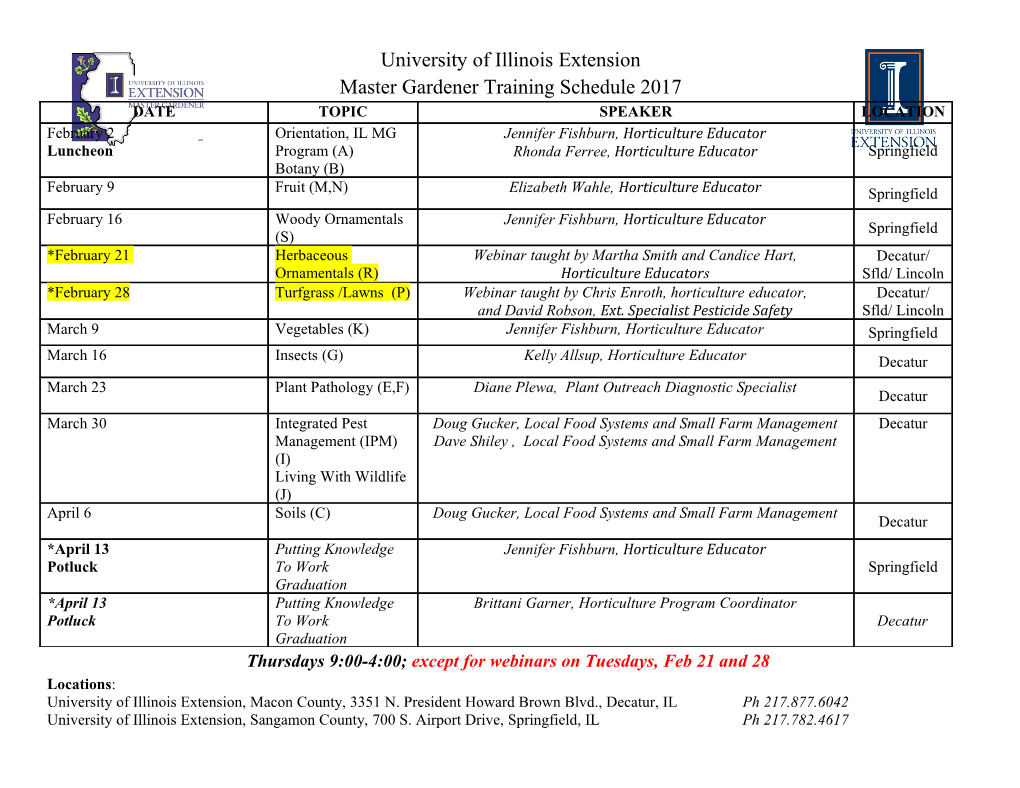
Magnetic Microtraps for Cavity QED, Bose-Einstein Condensates, and Atom Optics Thesis by Benjamin L. Lev In Partial Fulfillment of the Requirements for the Degree of Doctor of Philosophy California Institute of Technology Pasadena, California 2006 (Defended September 15, 2005) ii c 2006 Benjamin L. Lev All Rights Reserved iii To my parents, Daniel and Susan iv Acknowledgements One could not ask for a better advisor: throughout my years in graduate school, Hideo Mabuchi has been a friend as well as a mentor. His passion and dedication to science breathes life into research and inspires all who are fortunate enough to work with him. He has not only taught me how to build an experiment, but also, how to think as a scientist. I hope to honor his efforts by carrying his unceasing inquisitiveness wherever I go. In addition to Hideo, I credit Jakob Reichel with encouraging my development as a scientist. Jakob and his wife, Johanna, have been amazingly kind to me over the years, and I am grateful to both Jakob and Professor T. W. H¨ansch for allowing me to participate in their experimental efforts in Munich. Tilo Steinmetz, Philipp Treutlein, Peter Hommelhoff, and Yves Colombe have been wonderful labmates and hosts, and I thank them for teaching me to be a true M¨unchener. I feel fortunate to have been welcomed into Oskar Painter’s lab and appreciate his interest in my research and scientific development. It has been a joy to work with his students, Paul Barclay and Kartik Srinivasan. Hideo has assembled a group of students and colleagues second to none, and I feel privileged to have had the opportunity to work with them: Michael Armen, John Au, Andrew Berglund, Andr´eConjusteau, Andrew Doherty, JM Geremia, Salman Habib, Asa Hopkins, Chungsok Lee, Tim McGarvey, Kevin McHale, Anthony Miller, Benjamin Rahn, Jen Sokol, John Stockton, Ramon Van Handel, and Jon Williams. Grad school would not have been the same without the tomfoolery of Mike, Andy, Stockton, McKeever, and Noah Stern—truly the best partners-in-crime a guy could ever want. v Jeff Kimble and his students in the Caltech Quantum Optics group have been especially kind in their assistance throughout the years. David Boozer, Joseph Buck, Kevin Birnbaum, Jason McKeever, and Christoph N¨agerl deserve special thanks. The assistance of Axel Scherer, Michael Roukes, and their students and postdocs—notably Darrell Harrington and Eyal Buks—has been crucial. To all my LA, East Coast, and Georgenstraße friends, teachers, and family, I say thank you for encouraging me throughout the years. My mother and father have been both my inspiration and my anchor. Lastly, I want to thank my wife-to-be, Elizabeth, for her love and support. vi Abstract The system comprised of an atom strongly coupled to photons, known as cavity quantum electrodynamics (QED), provides a rich experimental setting for quantum information processing, both in the implementation of quantum logic gates and in the development of quantum networks. Moreover, studies of cavity QED will help eluci- date the dynamics of continuously observed open quantum systems with quantum- limited feedback. To achieve these goals in cavity QED, a neutral atom must be tightly confined inside a high-finesse cavity with small mode volume for long periods of time. Micro- fabricated wires on a substrate—known as an atom chip—can create a sufficiently high-curvature magnetic potential to trap atoms in the Lamb-Dicke regime. We have recently integrated an optical fiber Fabry-Perot cavity with such a device. The mi- crowires allow the on-chip collection and laser cooling of neutral atoms, and allow the magnetic waveguiding of these atoms to an Ioffe trap inside the cavity mode. Mag- netically trapped intracavity atoms have been detected with this cavity QED system. A similar experiment employing microdisks and photonic bandgap cavities is nearing completion. With these more exotic cavities, a robust and scalable atom-cavity chip system will deeply probe the strong coupling regime of cavity QED with magnetically trapped atoms. Atom chips have found great success in producing and manipulating Bose-Einstein condensates and in creating novel atom optical elements. An on-chip BEC has been attained in a miniaturized system incorporating an atom chip designed for atom interferometry and for studies of Josephson effects of a BEC in a double-well potential. Using similar microfabrication techniques, we created and demonstrated a specular vii magnetic atom mirror formed from a standard computer hard drive. This device, in conjunction with micron-sized charged circular pads, can produce a 1-D ring trap which may prove useful for studying Tonks gases in a ring geometry and for creating devices such as a SQUID-like system for neutral atoms. This thesis describes the fabrication and employment of these atoms chips in experiments at both Caltech and Munich, the latter in collaboration with Professors Theodore H¨ansch and Jakob Reichel at the Max Plank Institute for Quantum Optics. viii Contents Acknowledgements iv Abstract vi Preface 1 1 Introduction 23 2 Atom Chips 29 2.1 Introduction . 29 2.2 Microwire traps . 31 2.3 Zoology of microtraps . 34 2.3.1 The wireguide, U-trap, and Z-trap . 35 2.3.2 Variations: H-trap, dimple trap, P-trap, et cetera . 37 2.3.3 The Libbrecht-style Ioffe trap . 42 2.3.4 Permanent magnets and RF and electrostatic fields . 44 2.4 Atom chip loading . 45 2.4.1 Macro U-MOT . 48 2.4.2 D-MOT . 53 2.4.3 Sub-doppler cooling and optical pumping . 55 2.4.4 D-trap and rotation . 56 2.4.5 Z-trap transfer . 57 2.4.6 Imaging . 58 ix 3 Experimental Details 61 3.1 Vacuum system . 61 3.2 Atom chip assembly . 68 3.3 Laser system . 70 3.4 Computer control system . 76 4 Fabrication of Micro-Magnetic Traps for Cold Neutral Atoms 81 4.1 Fabrication Challenges and Constraints . 81 4.2 The elements of atom chip fabrication . 83 4.2.1 The photomask . 85 4.2.2 The substrate . 85 4.2.3 Substrate cleaning . 87 4.2.4 Thermal evaporation . 87 4.2.5 Photoresist spinning and baking . 89 4.2.6 UV exposure . 90 4.2.7 Developing . 90 4.2.8 Ozone dry stripping . 91 4.2.9 Wire contacts . 91 4.2.10 The mirror . 92 4.3 Specific fabrication techniques: wet etching, ion milling, lift-off method, and electroplating . 96 4.3.1 Wet etching and ion milling . 96 4.3.2 The lift-off method . 99 4.3.3 Electroplating . 100 4.4 Trap fragmentation . 104 4.5 Conclusion . 105 5 Cold Collisions and Cesium Microtrap Losses 106 5.0.1 Collision Basics . 107 5.1 Cold collision processes . 108 5.1.1 Excited-state collisions: MOT loss mechanisms . 109 x 5.1.2 MOT diffusion losses . 111 5.1.3 Background losses . 111 5.1.4 Ground-state loss mechanisms . 113 5.1.5 Majorana spin-flip losses . 115 5.2 Cesium microtrap losses . 116 5.2.1 U-MOT losses . 117 5.2.2 U-trap losses . 134 5.2.2.1 Background collisions . 134 5.2.2.2 Ground-state collisions and Majorana spin-flips . 135 5.2.3 Elastic collisions . 137 5.2.4 Concluding remarks . 138 6 The Atom-Cavity Chip: Combining microwire traps with photonic bandgap cavities and microdisks 140 6.1 Introduction . 140 6.2 Cavity QED with microcavities . 146 6.2.1 Photonic bandgap cavities . 146 6.2.2 Microdisks . 149 6.2.3 Comparison to other cavity systems . 151 6.3 Experimental proposal . 154 6.4 Single atom detectability using PBG cavities . 160 6.4.1 Single atom detection signal-to-noise . 163 6.4.2 Simulated atom transits and cavity induced force . 168 6.5 Single atom detectability using microdisk cavities . 174 6.6 Conclusion . 177 7 Fiber-Gap Fabry-Perot Cavity: The first atom-cavity chip 178 7.1 Introduction . 178 7.2 Experimental details . 180 7.2.1 Atom chip loading and waveguiding . 181 7.2.2 The fiber-gap cavity . 186 xi 7.3 Signal-to-noise and spontaneous emission analysis using the master equation . 191 7.3.1 Signal-to-noise and spontaneous emission . 193 7.3.2 Simulated atom transits and cavity induced force . 199 7.4 Experimental results . 201 7.5 Outlook . 208 8 Atom Mirror Etched from a Common Hard Drive 210 8.1 Overview . 210 8.2 Design and fabrication of the hard drive atom mirror . 212 8.3 Experimental details . 215 8.4 Results . 217 9 A 1-D Magnetoelectrostatic Ring Trap for Neutral Atoms 220 9.1 Overview . 220 9.2 Ring trap design . 221 9.3 Device electrical leads and trap perturbations . 224 9.4 Fabrication . 226 9.5 Trap loading and surface effects . 227 9.6 Discussion . 229 10 Splitting a BEC in a Magnetic Double-Well Potential: Atom inter- ferometry and Josephson effects on an atom chip 232 10.1 The double-well chip . 232 10.1.1 Josephson effects . 234 10.1.2 The magnetic double-well . 237 10.2 The glued-cell chamber . 243 A Magnetic Fields, Gradients, and Trap Minima of U- and Z-Traps 249 A.1 Infinitely thin linear wires . 250 A.2 Force on an atom in a U-trap . 251 A.3 U-trap minimum and gradients . 253 xii B Matlab Code 259 B.1 Cavity QED: transmission, signal-to-noise, and spontaneous emission 259 B.2 Master equation for a two-level atom . 263 B.3 Magnetic field, gradient, and curvature for U- and Z-traps and waveguides265 B.4 Computer control code for the atom chip experiment .
Details
-
File Typepdf
-
Upload Time-
-
Content LanguagesEnglish
-
Upload UserAnonymous/Not logged-in
-
File Pages325 Page
-
File Size-