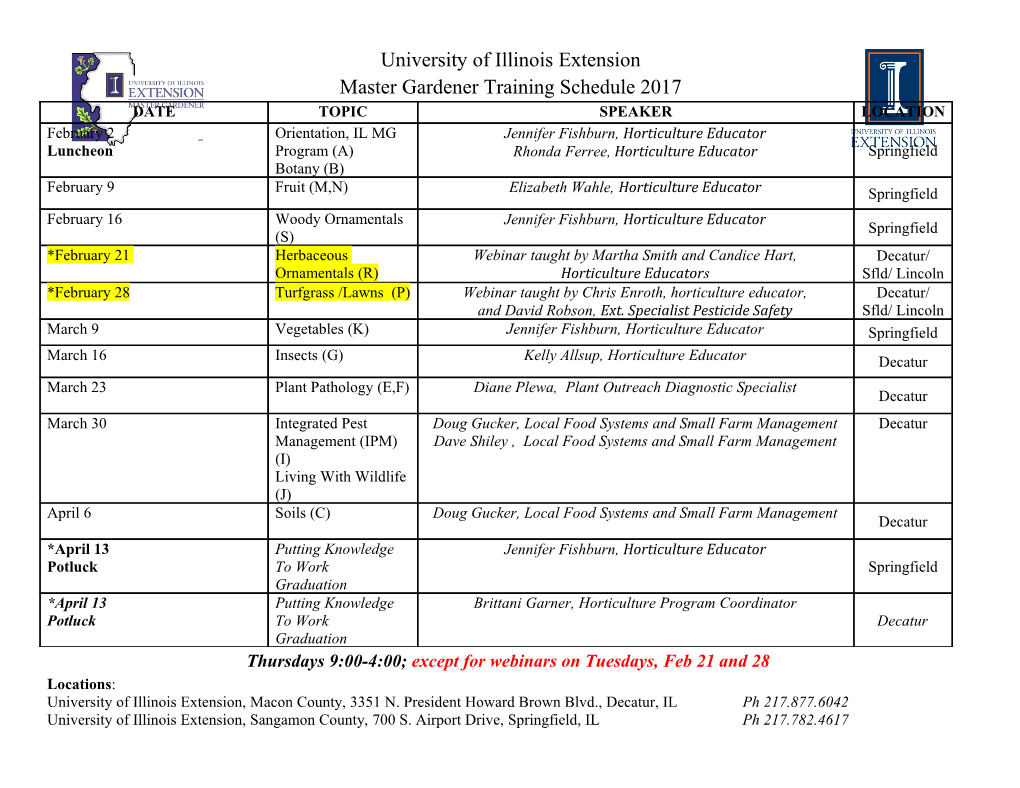
USING RESOURCES FROM NEAR-EARTH SPACE JOHN S. LEWIS University of Arizona DAVIDS. McKAY NASA Johnson Space Center and BENTON C. CLARK Martin Marietta Civil Space & Communications The parts of the solar system that are most accessible from Earth (the Moon, the near­ Earth asteroids, and Mars and its moons) are rich in materials of great potential value to humanity. Immediate uses of these resources to manufacture propellants, structural metals, refractories, life-support fluids and glass can support future large-scale space acitivites. In the longterm, non-terrestrial sources of rare materials and energy may be of great importance here on Earth. I. INTRODUCTION Space activities have become so much a part of modem life that they are almost invisible. We are aware how important communications satellite relays are for inexpensive long-distance telephone calls and television news broadcast, and we see the color images of Earth's cloud cover in the weather reports on TV. But we are usually not aware that the commercial airplane in which we fly or the ferry we ride locates itself by using navigation satellites 12,000 miles above our heads. We may never hear that the Soviet Union launched several satellites each year dedicated to assessing the American, Canadian, and Argentine wheat crops to help plan their grain purchases. We may be even more astonished to know that the truck we just passed on the highway is being tracked by a satellite, or that the strategic balance between the great powers has long been monitored based on photographic, electronic imaging, radar, infrared, and signal monitoring from space. Information from space gives us our first warning of preparations for war, the best guarantee against surprise attack. It also warns us of oil spills, crop disease, severe storms, ozone holes, forest fires, and climate change. Space is already worth roughly $100 billion per year to the global economy. [3] 4 J. S. LEWIS ET AL. In addition, the exploration of space plays a fundamental role in mod­ em culture. We daily glean new knowledge of the origin and evolution of planets, of the environment within which we live, and of the laws that govern global change. From our studies of other planets we bring home a greater understanding of Earth. We share in the excitement of discovery as human explorers explore alien landscapes. We share also in the traditions of western culture, in which experimentation and exploration constantly expand our hori­ zons and test the assumptions and dogmas of the past. The urge to test ideas, hypotheses, and assertions against objective standards is not only congenital in our culture; it is contagious. Where such testing is absent the true cannot be distinguished from the false; the tyrant can stand unchallenged; obsolete habit can successfully withstand innovative insight. In the emerging world of great-power cooperation and friendly competi­ tion, it is likely and highly desirable that one of the most important drivers of research and innovation, military spending, will diminish sharply in impor­ tance. But every highly industrialized country knows that technical innovation and improved productivity are the keys to economic health. Space stands out as one of very few areas of human endeavor that can not only satisfy the explorational urge and provide us the facts and insights we need to exercise our stewardship of Earth, but also keep the cutting edge of scientific and technological innovation razor-sharp. It is in the best interests of humanity to lower the cost of activities in space, if only to make our accustomed services more affordable. This can be done in part by lowering launch costs from Earth, and in part by building spacecraft on assembly lines instead of one at a time. But there is more to the economics of space than simply continuing with business as usual. This book deals in a broad, timely way with the next giant step in human use of space: to harness the energy and material resources of nearby space, not just to lower the cost of present space activities, nor even simply to make future large-scale space activities much more affordable, but actually to make use of these resources in the service of the greatest material needs of humanity. Because we are concerned with the near future, we concentrate almost solely on the resource potential of nearby bodies in space. We consider the Moon, the near-Earth asteroids, and Mars and its two moons, Phobos and Deimos. II. THE MOON A total of 56 spacecraft (out of 75 attempts) were sent to the Moon or its vicinity between the first lunar mission in 1959 (the Soviet Luna 1 flyby) and the last in 1976 (the Soviet Luna 24 robotic sample return mission), a period of about 17 years. This golden age of lunar exploration matured through a number of stationary robotic landers in the Luna and Surveyor series, several orbital photographic mapping missions in the Lunar Orbiter and Luna programs, three robotic sample returns (Luna 16, 20 and 24 ), and two robotic rovers (Lunokhod 1 and 2), to six human landings in the Apollo USING RESOURCES FROM NEAR-EARTH SPACE 5 program, which returned a total of 381. 7 kg of lunar materials. Despite the efforts of many to promote new flight programs, only a single lightweight mission, Japan's Muses A, has been sent specifically to the the Moon since 1976, a time interval roughly equal in duration to the entire golden age of lunar exploration in which those 56 missions were flown. The only large spacecraft to approach the Moon in these years is the Jupiter-bound Galileo mission, which flew by the Earth-Moon system in 1991 and late 1992 for reasons related to its trajectory to Jupiter, quite incidental to lunar science. What did we learn from the golden age of lunar exploration? Why did we quit going to the Moon for so long? What might justify a return to the Moon? As the data from these early missions acccumulated, and as the returned samples were analysed in detail, it became clear that the Moon was the scientific key to much of the early and intermediate history of the Solar System; in particular, to how Earth formed and evolved. The lunar samples probably have been better studied than any samples from anywhere else, including Earth. Such studies have led to the hypothesis of the formation of a lunar core, mantle, and crust, and of an early "magma ocean" from which the lunar highlands formed. The Moon was found to have had a complex volcanic history, with many kinds of basalts and trace-element-rich differentiates forming and extruding or intruding, principally during the first two billion years of lunar history. Extrusive volcanism on the Moon tapered off and apparently ceased some time between roughly two and three billion years ago. One crucial result of lunar sample studies is that we now know in great detail the physical properties and the chemical and mineralogical composition of a broadly diverse set of rocks and soils collected from nine sites on the near side of the Moon, a set that appears, based on remote sensing data, to be broadly representative of the entire near side. We do not, however, have global coverage of the Moon. Only a rather narrow band, most of it close to the equator, was covered by the orbital geo­ chemical mapping (gamma-ray spectroscopy) experiments carried out during the Apollo program. Although Earth-based multispectral mapping has contin­ ued to improve since the Apollo era, we still do not have direct chemical data on most of the Moon's surface. Thus the task begun by Apollo, to carry out complete geochemical mapping of the Moon, remains as unfinished business. This precise mission will be the central task of two proposed robotic orbiters which may fly as early as the mid-1990s. In addition, a series of robotic landers have been proposed for the middle to late 1990s. These landers will be used to confirm and calibrate the orbital remote-sensing data at specific landing sites. They will also provide detailed geochemical, mineralogical, and geophysical data on the subsurface, and help certify potential landing sites for human visits to follow around the end of the century. Visits to the Moon during the Apollo program were short and intense. No effort was made to use lunar materials to help support the crew or to provide anything other than scientific samples for return to Earth. In fact, lunar dust 6 J. S. LEWIS ET AL. was a major annoyance in mission operations: dust contamination of the space suits was so severe that it would have seriously limited any additional lunar surface activities beyond those limited tasks actually accomplished by the Apollo landings. When we again resume flying crews to the Moon, unlike Apollo, we will make major efforts to take advantage of lunar materials in a variety of ways to support the needs of the lunar outpost and its transportation system. We may also find ways to use lunar resources to help us return useful products from the Moon to Earth. Lunar materials can be used to support human activity at an outpost in a variety of ways. The earliest use of regolith or rocks may be for radiation shielding and for protection against blowing dust raised by ascent and descent rocket engines. Thermal isolation, heat storage, and ballast mass for cranes or trucks are other possible early uses of raw (unprocessed) lunar materials. Our early experiments with actual processing will probably be aimed at the extraction of volatiles, especially at oxygen production from lunar minerals.
Details
-
File Typepdf
-
Upload Time-
-
Content LanguagesEnglish
-
Upload UserAnonymous/Not logged-in
-
File Pages12 Page
-
File Size-