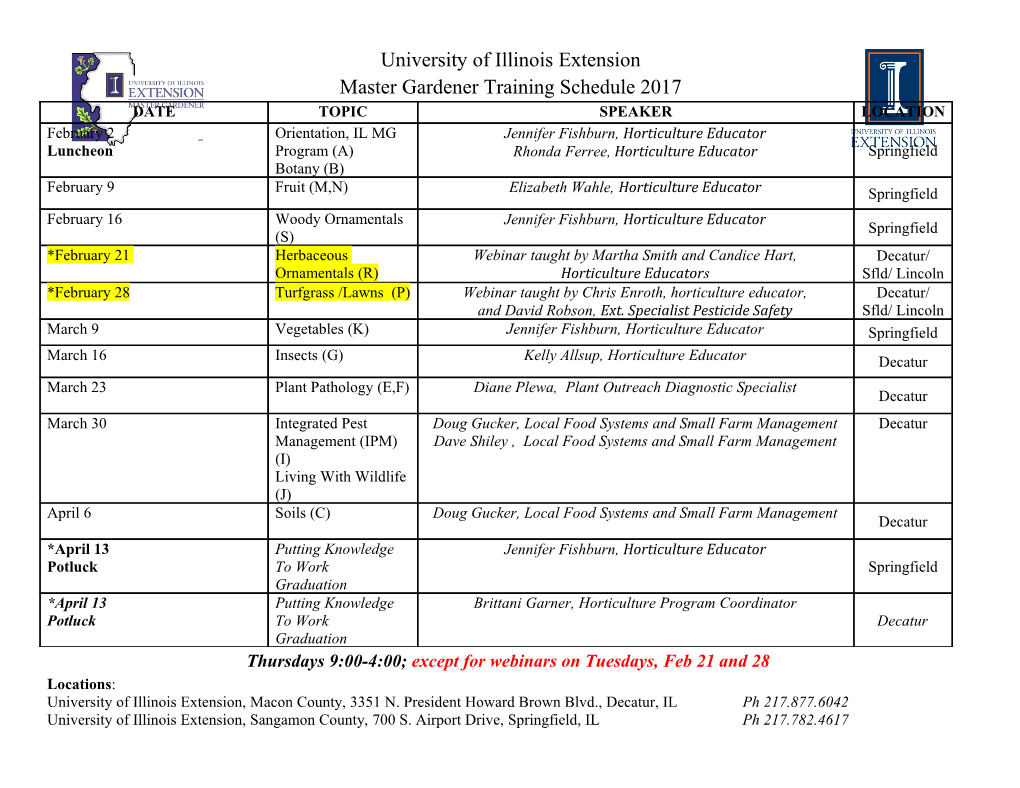
Design of Vehicle Structures for Crash Energy Management Contents Slide 2 of 80 Introduction • The final design was the product of a long evolution guided primarily by testing, supported by simple linear strength of material methods. • Tools include simple spring-mass models, beam element models, hybrid models and finite element models. • In recent years - demand from customers, regulators, and media to provide safer vehicles. 2 Contents Slide 3 of 80 Introduction • Current Design Practice - reviews the current modeling and design processes while identifying shortcomings for early design stages. • Crash/Crush Design Techniques for Front Structures - examines techniques for analyzing the front-end system and to compute the performance design objectives of subsystems and components. • Analytical Design Tools - introduces component design methods to create structural concepts for energy absorption and strength. • Vehicle Front Structure Design for Different Impact Modes - discusses strategy for designing structures for different frontal impact modes, including vehicle-to vehicle crashes. 3 Contents Slide 4 of 80 Current Design Practice • Lumped Mass-Spring Models • Limitations of LMS Models • Comparison Between LMS and FE-Based crashworthiness Processes Contents Slide 5 of 80 Current Design Practice • The Design process relies on calculating the crash pulse from either Lumped Mass-Spring (LMS) models or Finite Element (FE) models • Two tasks: – Vehicle body structure and major components packaged ahead of the front occupants, such as the power-train. – Designing the occupant environment such as the dummy, restraints and vehicle interior surfaces. Contents Slide 6 of 80 LMS based crash pulse estimation Figures from Priya Prasad , 2005 Contents Slide 7 of 80 FE Based Crash pulse Estimation Figures from Priya Prasad , 2005 Contents Slide 8 of 80 The LMS Model • Vehicle approximated by a one-dimensional lumped mass-spring system. • acceptable for modeling the basic crash features. • requires a user with extensive knowledge • Crush characteristics from static crush set- up. Figures from Priya Prasad , 2005 Contents Slide 9 of 80 Test set up Crush Test setup Kamal’s Lumped mass model Figures from Priya Prasad , 2005 Contents Slide 10 of 80 Acceleration vs Time Simulated acceleration histories with those obtained from the test, and it shows a very good agreement can be achieved Figures from Priya Prasad , 2005 Contents Slide 11 of 80 Side Impact LMS Model <#> of 78 Contents Slide 12 of 80 LMS Models Pros: • Quicker turnaround time – parametric studies at earlier design stages Cons: • One dimensional • Requires prior knowledge of spring characteristics Contents Slide 13 of 79 3D models Initial Results compare After 80 ms well with full scale crash tests Figures from Priya Prasad , 2005 Contents Slide 14 of 79 Frame Models and FE Models • To overcome 1D, 3D 3D frame simulation in MADYMO • The model also includes the power train external surfaces to accurately capture contact with FE model the barrier. Figures from Priya Prasad , 2005 Contents Slide 15 of 80 Basic Principles of Designing for Crash Energy Management •Match in 3D frame and FE model response for FOOTWELL intrusion •Difficult to reproduce by 1D LMS models Figures from Priya Prasad , 2005 Contents Slide 16 of 80 Crash/Crush Design Techniques for Front Structures • Designing for crash is multidisciplinary - very close interaction of – Biomechanics – Structures – Vehicle Dynamics – Packaging – Engineering analysis – Manufacturing Contents Slide 17 of 80 Basic Principles of Designing for Crash Energy Management Frontal offset Crash – 40 kmph Figures from Priya Prasad , 2005 Vehicle to vehicle crash – 70 kmph Contents Slide 18 of 80 Basic Principles of Designing for Crash Energy Management Desired Dummy Performance • Various occupant simulation models are used to study interactions between dummy, restraint system and the vehicle • A family of crash pulses or signatures that successfully meet specific injury criteria are defined. These pulses, in turn, define objective criteria for vehicle design • Desired crush sequence and mode will need to be selected and crush zones identified to assure the structural pulse • Several models are developed in parallel to study Contents Slide 19 of 80 Basic Principles of Designing for Crash Energy Management Stiff cage Structural Concept • Objective is to design a stiff passenger compartment structure • Structure should have a peak load capacity to support the energy absorbing members in front of it, without exhibiting excessive deformation (intrusion) Contents Slide 20 of 80 Basic Principles of Designing for Crash Energy Management Figures from Priya Prasad , 2005 Contents Slide 21 of 80 Basic Principles of Designing for Crash Energy Management Controlled Progressive Crush or Deformation With Limited Intrusion • Rear, roof, and side impact energy-absorbing structures deform upon direct impact in a mixed axial and bending mode • Bending dominant role – low energy content • In designs where light weight is desirable, axial mode will be a more appropriate candidate for energy absorption ContentsControlled Progressive Crush orSlide 22 of 80 Deformation With Limited Intrusion (contd) • Primary crush zone - relatively uniform, progressive structural collapse – Main energy absorbing structure- fore section of the power train compartment • Secondary crush zone -structural interface between the energy absorbing and occupant compartment structures – Avoid excessive load concentrations • Design strategy – soft front zone- to reduce the vehicle’s aggressivity in pedestrian-to- vehicle and vehicle-to-vehicle collisions – Two stiffer zones - primary and secondary. – Primary – main EA structure in the fore section – Secondary – structural interface between absorber and compartment • Extends to the dash panel and toe board areas Contents Slide 23 of 79 Design of structural topology / architecture • Based on ability to design in the crush mode for primary crush zones • Based on simplified 3D models • Once a skeleton is ready design loads can be estimated – Using Quasi Analytical studies – Sizing of thin shell components using computer codes. (ex. SECOLLAPSE) • Crush criteria will dictate the structural design. – NVH criteria and durability can be easily met if designed for crash. Model by Mahmood and Paluszny Figures from Priya Prasad , 2005 Contents Slide 26 of 80 3-D hybrid modelling of front end system • 3D hybrids and Lumped Mass collapsible beam elements used • Possible to analyze asymmetric impacts • The model grows progressively • The designed structures / sub-structures are readily analyzed in a system environment Finite Element Figures from Priya Prasad , 2005 Contents Slide 27 of 80 Evolving front-end system modelling LMS model LMS + FE model LMS + FE model ( increased FE) Complete FE with minimal LMS Figures from Priya Prasad , 2005 Contents Slide 28 of 80 Analytical Design Tools • Component Design – Column - axial load in compression – Beam - bending or combined bending and axial compression – Types of Deformations: • Buckling/collapse of a structural component is called local when the deformations (buckle/fold) are of local character and the collapse is confined to an isolated area of the component • It is global (an example is an Euler-type column buckling) when the whole component becomes deformed and subsequently collapses Contents Slide 29 of 80 Analytical Design Tools • Two Issues – Collapse Modes for Absorption of the kinetic energy of the vehicle • Axial • Bending – Crash resistance or strength to sustain the crush process and/or maintain passenger compartment integrity Contents Slide 30 of 80 Axial / Bending Mode • Axial – Most Effective – Difficult to achieve • Bending – Local hinges are formed – Structures designed for axial mode can also fail in this mode <#> of 78 Contents Slide 31 of 80 Axial Collapse – Mathematical Modelling • purely analytical / strictly experimental approaches • Analytical – Modeling mechanics and kinematics of folding – Assumptions were limiting – Average or mean crush load estimated from the energy balance – by equating the external work done by the crush load with energies dissipated in different types of deformation mechanisms in a folding process Figures from Priya Prasad , 2005 Contents Slide 32 of 80 Axial Collapse 1/3 -1/3 Pm = 38.27 Mo C t • where – Pm is the mean/average crush force 2 – Mo = σo t / 4,the fully plastic moment, – σo, is the average flow stress (σo = (0.9 to 0.95) σu), – σu is the ultimate tensile strength of the material, – C = 1/2 (b+d) with b and d being the sides of a rectangular box column, – t its wall thickness Contents Slide 33 of 79 Variation of structural effectiveness – Experimental results • = specific energy (SE) / specific ultimate strength (SUS) • SE = maximum energy / weight • SUS = US / density • = material volume / total volume Plot for square and rectangular thin wall columns Figures from Priya Prasad , 2005 Contents Slide 34 of 80 Axial Collapse Experimental: • The expression for mean crush load is obtained from the expression for specific energy (Es=Pm/ρφAo) and is of the form: Pm = η σu φ Ao • ρ being the density and Ao the overall area of the section as defined by its outer circumference Contents Slide 35 of 80 Mahmud and Paluszny • Local buckling at critical loads • Leads to collapse and subsequent folding • For low t/b ratios – Large irregular folds – Crumpling – Global Buckling or – Bending type instability Folding pattern of thin-walled box with very small thickness/width
Details
-
File Typepdf
-
Upload Time-
-
Content LanguagesEnglish
-
Upload UserAnonymous/Not logged-in
-
File Pages85 Page
-
File Size-