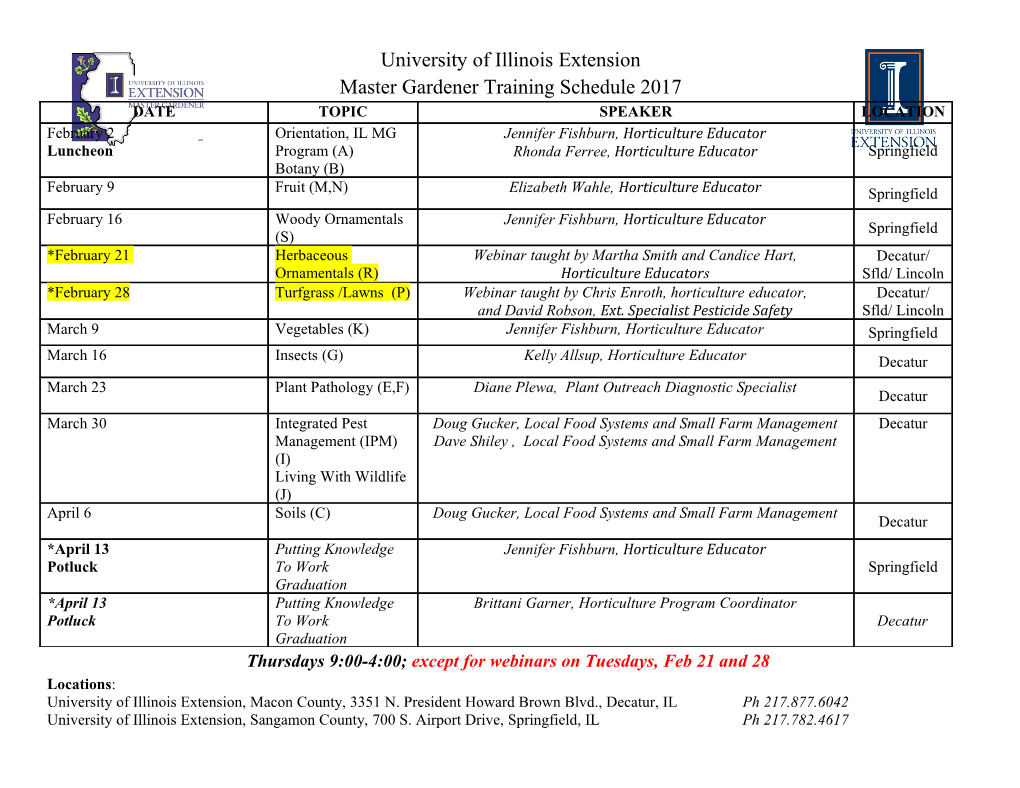
Aromatics in Synthesis Alan Spivey [email protected] Format and scope of presentation • Formation of metal aryls: – Reductive metalation (Barbier conditions) – Halogen-metal exchange (halogen-lithium and Grignard metathesis) – Deprotonation [directed ortho-lithiation (DoM)] • Transmetalation & cross-coupling reactions: – Transmetalation to Cu, Zn, Sn, B, Ge, & Ce – Kumada-Corriu, Negishi, Stille, Suzuki, Hiyama/Denmark, Heck, Sonogashira & sp3 • Buchwald/Hartwig amination & etherification: – Amination of aryl chlorides – Biaryl ether formation • Birch reduction: – Reduction/alkylation • 'De novo' aryl synthesis reactions: – Fischer carbene chemistry (Dötz reaction) – Cobalt mediated [2+2+2] reactions (Volhardt reaction) – Ring Closing Metathesis (RCM) Utility of aryl metals - overview Ar R Bn R Kumada-Corriu R HO R Kharasch R Negishi R R Suzuki O BnBr Suzuki Stille Stille Sonogashira R Negishi R OH M Heck, Suzuki M' RCHO transmetalate Stille, Negishi R R R R M = Li, MgX, ZnX cyanation M' = ZnX, SnR3, SiX3, BR2 CN DMF CHO boronate Baeyer-Villager Buchwald/Hartwig amination & R R RCN CO2(s) etherification COR CO2H OH OAr/R NR2 Beller Angew. Chem Int. Ed. 2003, 42, 1661 (DOI) R R R R R Reductive metalation • By using an aryl halide and an activated free metal (Mg, Li, Zn) – Review: Yus Tetrahedron 2003, 59, 9255(DOI); Clayden ‘Organolithiums: Selectivity for Synthesis’ 2002 (Pergamon) Mg(m), Et2O, Δ Ar-Mg-X Li(m), THF, Δ NB. alloys with 1-2% sodium often Ar-X Ar-Li employed and/or metals with high surface area e.g. 'Reike metals' X = Cl, Br, I Zn, THF, Δ Ar-Zn-X – Mechanism: Single Electron Transfer (SET): Andrieux J. Am. Chem. Soc. 1986, 108, 638 (DOI) Ar-X + Mg Ar-X + Mg Ar + Mg-X Ar-Mg-X Ar-X + Li Ar-X + Li Li + Ar + Li-X Ar-Li + Li-X – Features: • Wurtz coupling a side reaction particularly for iodides and bromides • Requires elevated temperatures (i.e. >25 °C) – e.g. for pyridines (using Barbier in situ electrophile quenching) • Sugimoto Tet. Lett. 2002, 43, 3355 (DOI)& J. Org. Chem. 2003, 68, 2054 (DOI) Mg* (4eq), THF, rt 30min OH 2-I [67%] I Mg* = activated Mg by reduction of 3-I [73%] N Me t MgCl2 by Li and naphthalene in THF Me O (3eq) N Bu 4-I [64%] Me Reductive metalation – zincation promoted by LiCl • reductive zincation promoted by LiCl: – Knochel Angew. Chem. Int. Ed. 2006, 45, 6040 (DOI) Zn·LiCl (1.4eq),THF,RT Cl 10 min O [94%] I S I I S ZnI·LiCl I S CuCN.2LiCl O NB. NO zincation using Zn (m) alone Zn·LiCl (1.4 eq), THF, RT 10 min Br S CuCN.2LiCl ClLi·IZn S O [87%] O • ortho-directing affect of FGs – esters, ketones, aryl sulfonates, acetates, carbamates, triazenes: – Knochel J. Am. Chem. Soc. 2007, 129, 12358 (DOI) I I 5 I Zn·LiCl (1.15 eq), THF, RT Cl tBu 12 h O 7 tBu I N N LiCl·IZn N OTs O OTs [78%] OTs Halogen-metal exchange - overview • By exchange of an aryl halide with an alkyl metal (discovered by Gilman & Wittig) – reviews: Schlosser Synlett 2007, 3096 (DOI) – Hal-M exchange and deprotonation of heterocycles; Knochel Chem. Comm. 2006, 583 (DOI); Schlosser Angew. Chem. Int. Ed. 2005, 44, 376 (DOI); Yus Tetrahedron 2003, 59, 9255(DOI); Clayden ‘Organolithiums: Selectivity for Synthesis’ 2002 (Pergamon); Knochel Chem. Eur. J. 2000, 6, 767 (DOI) NB. use of 2 equiv of t-BuLi common. Irreversibility ensured by 2nd equiv. eliminating X- from t-BuX: SBuLi, THF iPrMgBr, THF Ar-Li Ar-Mg-Br Ar-Br t-BuLi Ar-X Ar-Li st s i 1 eq BuBr PrBr + t-BuLi ++ LiX t-BuX 2nd eq – Mechanism: depends on structure, for aryls halophilic displacement likely: • Driven by thermodynamics: sp3 to sp2 anion Ar Ar-Br + R M Br M Ar-M + RBr R possible intermediate ate complex • Initial complexation of the alkyl metal to the halide supresses competitive addition – Features: • Proceeds at low temperature (e.g. -100 °C); supresses addition to C=N groups & deprotonation • Rate of exchange: Ar-I > Ar-Br > Ar-Cl >> Ar-F • Solvent dependent selectivity due to aggregation & Schlenck equilibrium (Grignard reagents): 2 Ar-Mg-X Ar-Mg-Ar + X-Mg-X Halogen-Mg exchange • iPrMgBr at -40°C: – indole synthesis: Knochel Org. Lett. 2002, 4, 1819 (DOI) Y 1) iPrMgBr, THF, -20°C Y Y H NNMe2 2) CuCN·2LiCl NNMe2 H3O N Me X I 3) OMe X Br X MeO X = CO2Et, Y = I [90%] X = CO2Et, Y = I [75%] X = NC=NMe2, Y = H [71%] X = NC=NMe2, Y = H [68%] – one-pot Mg-halogen exchange / Kharasch cross-coupling: – Quéguiner Tetrahedron 2002, 58, 4429 (DOI); Knochel Synlett 2002, 1008 (DOI) 1) iPrMgCl, THF, -40°C I EtO2C 2) Pd(dba)2 cat. dppf, -40°C, 6h CN N EtO2C [87%] CN NCl Halogen-Mg exchange – advances • LiCl acceleration: iPrMgCl·LiCl participates in Br-Mg exchange faster than iPrMgCl itself: – Knochel Angew. Chem. Int. Ed. 2004, 43, 3333 (DOI) & Org. Lett. 2004, 6, 4215 (DOI) & Chem. Commun. 2005, 543 (DOI); & Synlett 2007, 980 (DOI) & Chem. Commun. 2007, 2075 (with free carboxylic acids) (DOI) – e.g. Knochel Chem. Commun. 2006, 726 (>998:1 regioselectivity) (DOI) iPrMgCl·LiCl Br Br Br MgCl Br E THF, -30 °C, 2 h E N OTs N OTs N OTs [95%] 8 examples [71-93%] • chelating diamine supresses addition reactions – pyrimidine I-Mg exchange: – Wang Org. Lett. 2006, 8, 3141 (DOI) H H N N H N iPrMgCl N N N ClMg ClMg N THF, 0 °C ClMg ~70% ~20% ~10% N I N + O N Cl ~exclusively via Mg N N ClMg H N N ClMg Halogen-Zn exchange i • using Pr2Zn & Li(acac) (presumed to form ‘ate’ complex): – also tolerates ketones and aldehydes – Knochel Angew. Chem. Int. Ed. 2004, 43, 1017 (DOI) • using Et2Zn & a phosphazine base (tBu-P4) as catalyst: – tolerates esters – Kondo Chem. Commun. 2006, 3549 (DOI) Halogen-Cu exchange • even wider functional group tolerance: – using lithium di(neopentyl)cuprates - tolerates ketones and aldehydes • Knochel Angew. Chem. Int. Ed. 2002, 41, 3263 (DOI) I 1) Neopent2CuLi, THF, -40-0°C [80%] OO 2) allyl bromide Neopent – using lithium di(neophyl)cuprates – tolerates highly functionalised indoles • Knochel Org. Lett. 2004, 6, 1665 (DOI) & Chem. Commun. 2006, 2486 (DOI) I I 1) Nphyl2CuLi, 25°C Ph I N 2) DMAP cat., PhCOCl N O SO2Ph [84%] SO2Ph 1) Nphyl2CuLi, -78°C 2) COCl [65%] 1) NH2NH2.H2O Nphyl 2) EtOH, Δ O N O [88%] N N N Ph H Ph SO2Ph Deprotonation – overview • Reviews: Schlosser Synlett 2007, 3096 (DOI) – Hal-M exchange and deprotonation of heterocycles; Schlosser Angew. Chem. Int. Ed. 2005, 44, 376 (DOI); Clayden ‘Organolithiums: Selectivity for Synthesis’ 2002 (Pergamon). • Deprotonation of benzene derivatives: – thermodynamically possible for alkyl metals (pKa BuH ~45, pKa ArH ~38), but kinetically slow – no regiocontrol (without directing groups) • Deprotonation of aromatic heterocycles (ortho- to ring heteroatoms): – Thermodynamically more favourable (pKa ArC=NH ~35) and kinetically faster than for benzene particularly: • ortho to ring N • ortho to directing substituents (DoM – see later) DG DG BUT: inductive and chelative pair-pair stabilisation stabilisation electron N Li N Li N Li repulsion – Kinetics: due to: DG DG Complex Induced inductive acidification and Li Proximity Effects H of ortho-protons R # NNH stabilising TS – Low temperatures & bulky bases required to supress addition reactions to C=N function: • Quéguiner Tetrahedron 2001, 57, 4059 (DOI) RLi as RLi as nucleophile base + RH (g) N R addition N lithiation N Li Li Thermodynamic vs kinetic deprotonation (Li) • thermodynamic deprotonation using hindered lithium/magnesium amide bases: – amine anions are poorly nucleophilic and undergo slow competitive addition reactions – reversible equilibration, success depends on the pKa of the ortho-proton being lower than that of the amine: LiTMP > LDA ArH + R2NLi ArLi + R2NH pK 37.3 N N a pKa 35.7 reversible Li Li • kinetic deprotonation using alkyl lithium bases (RLi): – branched alkyl lithiums undergo slow competitive nucleophilic addition – irreversible loss of RH, maximum basicity of alkyl lithiums in non-co-ordinating solvents e.g. hexane with TMEDA co-solvent – review (structures of lithium complexes): van Koten Angew. Chem. int. Ed. 2005, 44, 1448 (DOI) Me2 Me2N Me2 ArH + RLi ArLi + RH (g) N Li N Li ~ ~ Li pKa ~45 irreversible N Me2N Me2 Me2N • mechanisms - disputed: – acidity due to two factors: i) inductive activation, and ii) Complex Induced Proximity Effects (CIPE) – review: Snieckus & Beak Angew. Chem. Int. Ed. 2004, 43, 2206 (DOI); see also Collum J. Am. Chem. Soc. 2000, 122, 8640 (DOI) & J. Am. Chem. Soc. 2007, 129, 2259 (DOI); Mortier Org. Lett. 2005, 7, 2445 (excellent discussion of specific case of DoM of m- anisic acid) (DOI) Thermodynamic vs kinetic deprotonation (Li) • regioselectivity: kinetically and thermodynamically most acidic protons may differ: – Fort J. Org. Chem. 2003, 68, 2243 (DOI); J. Org. Chem. 2002, 67, 234 (DOI); Org. Lett. 2000, 2, 803 (DOI) 1) LDA (1eq) 1) BuLi-LiDMAE (3eq) 3 TMS THF, -78°C hexane, -78°C 6 2) TMS-Cl 2) electrophile NCl N Cl E NCl thermodynamic kinetic deprotonation deprotonation Electrophile E yield NMe MeI MeI 70 HO 2 I2 I 80 BuLi (2eq) t-BuCHO CH(OH)t-Bu 90 THF MeCOEt MeC(OH)Et 60 -78°C -> 25°C DMF CHO 15 MeSSMe SMe 92 ClBu Sn SnBu 84 O N .BuLi 3 3 Li BuLi-LiDMAE Directed ortho-metalation (DoM, Li) • directed metalation groups (DMGs) attached via carbon can assist ortho-metalation – Reviews: Snieckus & Beak Angew. Chem. Int. Ed. 2004, 43, 2206 (DOI); Sniekus J. Organomet. Chem. 2002, 653, 150 (DOI); Quéguiner J. Het.
Details
-
File Typepdf
-
Upload Time-
-
Content LanguagesEnglish
-
Upload UserAnonymous/Not logged-in
-
File Pages60 Page
-
File Size-