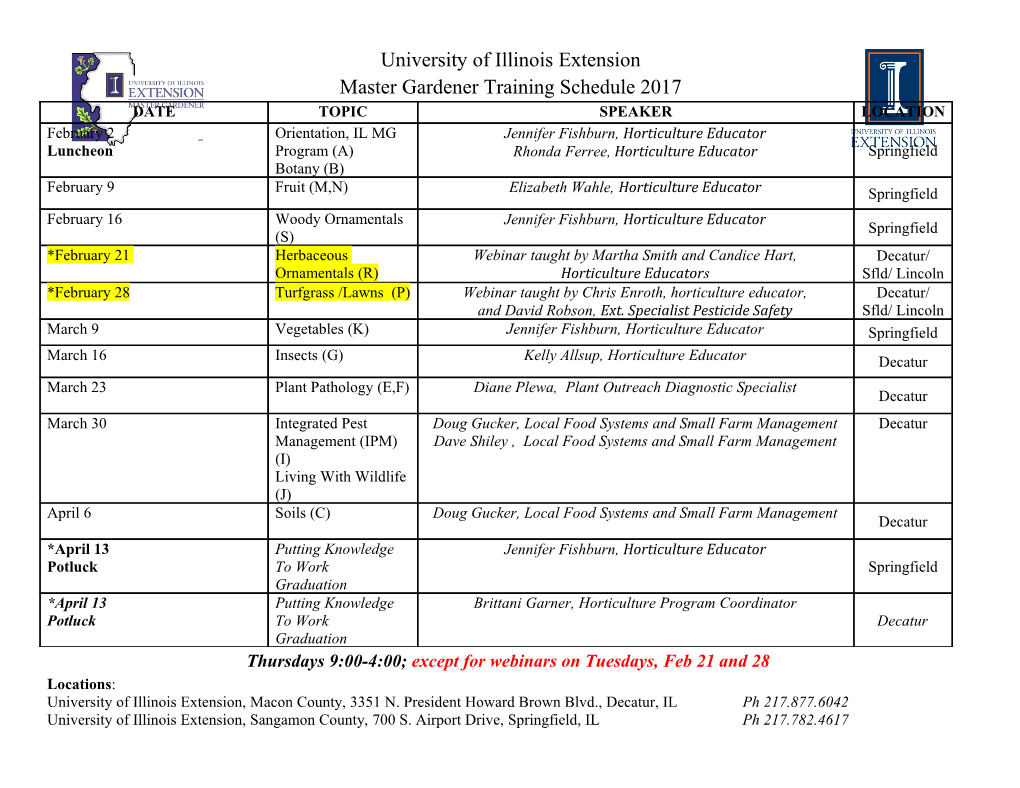
464 Core-directed protein design Derek N Woolfson For various reasons, it seems sensible to redesign or design and combinatorial design. I discuss the latter in detail later. proteins from the inside out. Past approaches in this field have By wholly rational design, I mean the direct application of involved iterations of mutagenesis and characterisation to sequence-to-structure rules to achieve a specific target ‘evolve’ designs. Increasingly, combinatorial approaches are structure. Preferably, the rules should be understood in being taken to select ‘fit’ sequences from libraries of variant physicochemical terms. The rules may be positive, that is, proteins. In particular, in silico methods have been used to to design towards the target, or negative, to disfavour and good effect. More recently, experimental methods have been design away from alternative structures [4–6]. developed and improved. We are now in a position to redesign stability and function into natural protein frameworks confidently Not surprisingly, current successes in wholly rational and to attempt de novo designs for more ambitious targets. approaches are limited to special cases. From the perspective of core-directed design, the best examples are the rules for Addresses oligomer-state selection in coiled coils, which are two-, Centre for Biomolecular Design and Drug Development, School of three-, four- or five-stranded helical bundles. The seminal Biological Sciences, University of Sussex, Falmer BN1 9QG, UK; e-mail: [email protected] studies of Harbury et al. [7] on mutants of the leucine zipper show that the oligomer state can be distinguished — at Current Opinion in Structural Biology 2001, 11:464–471 least between parallel dimer, trimer and tetramer — using 0959-440X/01/$ — see front matter appropriate combinations of isoleucine and leucine at © 2001 Elsevier Science Ltd. All rights reserved. the a and d positions of the abcdefg (heptad) sequence Abbreviations repeat. The resulting rules have been, and doubtless will PDB Protein Data Bank continue to be, improved [1,8,9]. Nonetheless, the current TIM triose phosphate isomerase rules provide clear guidelines for constructing specified WT wild-type coiled-coil oligomers and form the basis of more ambitious designs [1,10•,11–14]. Introduction The organisation of a hydrophobic core provides the main Iterative experimental design processes driving force for protein folding and stabilisation and, in For non-coiled-coil proteins, design is not so prescribed some cases, native-state specification. It seems reasonable, and alternative routes to correctly folded, stable structures therefore, to design new proteins from the inside out. are needed. One approach is to design iteratively, adding Increasingly, protein designers are taking this approach, small positive and negative design features step-by-step which I refer to as core-directed design. and testing the intermediates experimentally. In certain cases, core-directed design is protein design The design of α3D illustrates this process [15]. α3D is a single- heaven; for example, stability and specificity can be built chain protein designed to form a mixed parallel/antiparallel into simple coiled-coil structures using a few knowledge- three-helix bundle. The starting point is Coil-Ser, a based rules that can be applied without involving previously described three-stranded coiled coil [16]. This computers [1]. Unfortunately, this understanding does not is used as a template to design α3C [17], which has extend to globular proteins. Early attempts to design shortened helices, helix-capping motifs and a repacked globular proteins took iterative approaches, in which related hydrophobic core to introduce heterogeneity and disfavour sequences were sequentially tested for stability and coiled-coil-type packing. The NMR structure of α3D (a structural uniqueness — effectively evolving the designs. variant of α3C) agrees reasonably with the design model. A Now, combinatorial methods are being applied. In these noteworthy point is the use of negative design: interhelix approaches, many core sequences that are potentially electrostatic interactions are used to orientate the helices in compatible with a target structure are tested simultaneously an anticlockwise manner and disfavour alternative topolo- and winners selected. Selection can be done in silico or via gies — this principle also works in a canonical coiled-coil wet experiments; the latter are generally referred to as system [10•,18]. The iterative redesign and characterisation directed-evolution methods. The main computational of α3D is ongoing [19]. Incidentally, Coil-Ser has been methods are amply reviewed elsewhere [2,3]. This review used as a template to make another single-chain three- focuses largely on recent experimental approaches to the helix bundle [20]. problem of core-directed design. Many iterative designs and redesigns have focused on Rule-based or wholly rational design and four-helix bundles, which offer a step up in complexity special cases from coiled coils. The classic example is DeGrado’s evolution It is convenient to consider two broad approaches in protein of four-helix-bundle designs, which was recently reviewed redesign and de novo design; namely, wholly rational design by Hill et al. [6]. Core-directed protein design Woolfson 465 Figure 1 (a) (b) (c) (d) Current Opinion in Structural Biology Orthogonal views of various four-helix-bundle structures. (a) WT ROP (PDB code 1rop; [60]). (b) The Ala2Ile2-6 core mutant of ROP (PDB code 1f4m; [27•]). (c) The A31P mutant of ROP (PDB code 1b6q; [28•]). (d) The de novo design α2D (PDB code 1qp6; [29]). Different chains of each structure are coloured blue and red; N termini are highlighted black. Gibney et al. [21] describe an iterative approach to map out The four-helix bundle ROP is a dimer of an antiparallel sequence space and the associated free-energy landscape helical hairpin (Figure 1a). The active RNA-binding site is of a previously designed four-helix-bundle maquette [22,23]. on the face formed by the two copies of helix 1, which are This is done on a modest scale, limited by the level of antiparallel; this provides a convenient probe for the native characterisation undertaken. The parent peptide has structure. The structure has a core of a and d layers from histidine at two a sites to promote haem binding and three coiled-coil-like heptad repeats. Regan’s group [26] has leucines at d sites. The apoform is unstable to guanidine systematically repacked this core. For example, in denaturation and displays structural heterogeneity. In an Ala2Leu2-6, the middle six a and d sites are exchanged for attempt to improve this design, single, double and triple alanine and leucine, respectively. Theoretically, this mutant mutants were made to introduce isoleucine, valine and maintains the wild-type (WT) core volume. Consistent phenylalanine at the d positions. The most-promising with this, the mutant is active but thermally stabilised. • mutants (in terms of stability and structural uniqueness) at Willis et al. [27 ] characterise a related mutant, Ala2Ile2-6, each stage are taken to the next stage; the first and second which is also thermally stabilised but inactive. The crystal iterations returned improved designs, but the third iteration structure explains the loss of activity: compared with the was disappointing as the peptides lost conformational WT structure, one protomer in the mutant is rotated 180° uniqueness. The maquette is being used as a template for around the dimer interface (Figure 1b). In this new topology, the iterative redesign of haem-binding pockets [24]. the two copies of helix 1 are juxtaposed diagonally rather than adjacent, which splits the active face. Interesting but cautionary tales from the repacking of four-helix bundles A more perplexing structural rearrangement occurs in the As highlighted for the coiled coils [1,7,25], repacking a ROP mutant A31P [28•], which is a helical dimer with hydrophobic core can have consequences other than changing reduced stability but some activity. The crystal structure stability and conformational heterogeneity. Four-helix reveals a remarkable architectural transformation to a bundles also alter in response to mutation. ‘bisecting U’ motif, in which the helical hairpins intercalate 466 Engineering and design to form a right-handed four-helix bundle (Figure 1c). The from high-resolution structures of the WT and mutant term ‘bisecting U’ is introduced by Hill to describe the proteins as the weighted sum of the atom–atom contacts structure of the designed four-helix bundle α2D (Figure 1d) within that region. Two weightings are included for [6,29]. Presumably, ROP A31P retains activity because the distance and solvent accessibility, which dampen contributions two copies of helix 1 remain adjacent, although they are from distantly spaced atoms and from surface-exposed ∆ parallel and not antiparallel as in the WT structure. This residues, respectively. nh is the difference between the dramatic rearrangement is particularly worrisome because it nh of the mutant and WT structures. Given the limited ∆∆ ∆ results from a single amino acid change to the ROP sequence. experimental data available, G and nh correlate reason- ∆∆ ∆ ably, and the slopes of G versus nh plots essentially Thus, simple rules like those for packing coiled coils are not provide values for the energy cost per contact lost. In this ∆ as forthcoming in ROP and similar systems. This probably respect, nh may prove useful in quickly
Details
-
File Typepdf
-
Upload Time-
-
Content LanguagesEnglish
-
Upload UserAnonymous/Not logged-in
-
File Pages8 Page
-
File Size-