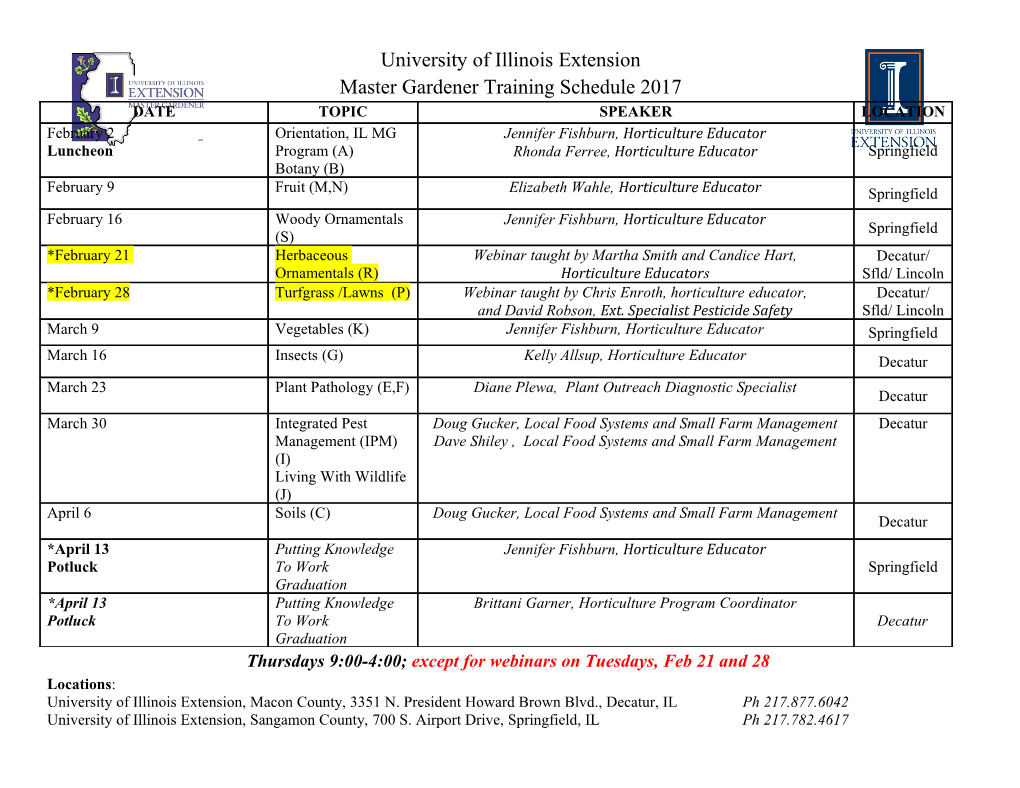
Updated manuscript Click here to access/download;Manuscript;thomas_bela_ceas_v2.docx Click here to view linked References The BepiColombo Laser Altimeter (BELA) – A Post-Launch Summary 1 2 Nicolas Thomasa, Hauke Hussmannb, Luisa M. Larac 3 a 4 Space Research and Planetology Division, University of Bern, Sidlerstrasse 5, CH-3012 Bern, Switzerland; b c 5 German Aerospace Center, Institute of Planetary Research, Planetary Geodesy, Berlin, Germany; Instituto 6 de Astrofísica de Andalucía, Granada, Spain 7 8 9 ABSTRACT 10 11 We provide a brief description of the BepiColombo Laser Altimeter (BELA) experiment for the joint ESA-JAXA mission 12 to Mercury, BepiColombo. The text describes the main elements of the instrument and discusses several of the problems 13 encountered during the instrument development. The actions taken to mitigate the issues are also described and the 14 resulting performance of the instrument as determined by combining ground test data with models of Mercury and the 15 spacecraft orbit is presented. The instrument has met the requirements set in 2005 and should provide a solid data set for 16 the global study of the topography and internal structure of Mercury 17 18 Keywords: BepiColombo, BELA, laser altimeter 19 20 21 1. INTRODUCTION 22 23 The European Space Agency’s BepiColombo mission to Mercury [1] was launched on 20 October 2018 from Kourou. The 24 stack comprised two spacecraft and a transfer module. The transfer module (MTM) uses solar-electric propulsion to bring 25 the two spacecraft to Mercury orbit. Arrival is foreseen in December 2025. The two spacecraft are the Mercury 26 Magnetospheric Orbiter (MMO) which has been built by the Japanese space agency, JAXA. The Mercury Planetary Orbiter 27 (MPO) has been built by ESA. 28 Onboard the MPO is the first European-built laser altimeter for interplanetary flight, the BepiColombo Laser Altimeter 29 (BELA). The instrument was selected in 2005 and the development has overcome a number of challenges in building this 30 type of system within the given constraints. The original requirements were that the instrument should weigh <12 kg and 31 consume <40 W while acquiring altimetry data at <2 m resolution from a 400 km x 1500 km orbit on a nadir-pointed 32 spacecraft. The final mass of the instrument was 14.2 kg and the power consumption is <30 W in nominal operation. 33 34 This paper briefly describes the investigation, the instrument, and the test performance in the laboratory. In-flight 35 commissioning so far suggests that the ground performance has not been modified during the launch phase. 36 37 38 1.1 Aims of the investigation 39 40 BELA is a joint Swiss-German project with smaller involvements from Spain and France. The scientific objectives of the 41 experiment are to measure 42 ★ the figure parameters of Mercury to establish accurate reference surfaces 43 44 ★ the topographic variations relative to the reference figures and a geodetic network based on accurately measured 45 positions of prominent topographic features 46 47 ★ the tidal deformations of the surface 48 ★ the surface roughness, local slopes and albedo variations, also in permanently shaded craters near the poles 49 50 51 BELA forms an integral part of a larger geodesy and geophysics package, incorporating radio science and stereo imaging. 52 The synergy between the instruments will allow determination of the planetary figure and gravity field, investigation of 53 the interior structure, study of Mercury’s surface morphology and geology, and possibly provide measurements of tidal 54 55 56 57 58 59 60 61 62 63 64 65 deformations. The offset between the centre of mass and the centre of figure will be derived. The reference surfaces and 1 the geodetic network will provide the coordinate system for any detailed geological, physical, and chemical exploration of 2 the surface. The topography is needed to develop digital terrain models which will allow quantitative study of the geology, 3 tectonics, and age of the surface. The topography is further needed for a reduction of the gravity field data because 4 topographical contributions to gravity must first be removed before using gravity anomalies for the investigation of sub- 5 surface structures. The use of topography together with gravity data will constrain, by an admittance analysis between the 6 two and with the help of a flexure model for the lithosphere, both lithosphere and crust properties. Examples here would 7 include the lithosphere elastic thickness (essential for the reconstruction of the thermal history of Mercury) and the crustal 8 density (essential for the construction of a Hermean internal model). In addition to the moments of inertia which will be 9 provided by the radio science experiment, the tidal deformations measured by BELA and the radio science instrument will 10 place further constraints on global models of the interior structure. BELA will contribute by providing the deformation of 11 the surface while the radio science package will measure the mass concentrations. Under favourable conditions, it will 12 even be possible to constrain the rheology of the interior of the planet by measuring the time lag between the motion of 13 the tidal bulge and the disturbing potential. 14 It is to be noted that the MESSENGER Laser Altimeter (MLA) onboard NASA’s MESSENGER spacecraft has provided 15 laser altimetry data at Mercury. However, the eccentric orbit of the spacecraft resulted in good quality data being acquired 16 only in the northern hemisphere. The southern hemisphere of Mercury is almost completely unexplored with altimetry. 17 Hence, BELA is likely to make a major contribution to Mercury studies well beyond the MESSENGER contribution. 18 19 The main observations to be performed are itemised in Table 1. 20 21 Table 1 Main observation types of BELA mapped to the science goals 22 23 Observation Goal 24 Type 25 Dense global grid of range measurements between S/C and Mercury’s surface to be 1 26 carried out throughout the whole science mission phase 27 28 2 Measure the topography of selected morphologic features 29 Measure periodic surface deformation as a function of time (i.e. as a function of true 3 30 anomaly of Mercury in its orbit around the Sun) by detecting periodic variations 31 32 4 Assist in determining Mercury’s pole position and rotation rate 33 Assist in determining periodic variations of Mercury’s rotation rate (physical 5 34 librations) 35 Measure the albedo globally (at laser wavelength of 1064 nm) actively (with laser 6 36 pulse) including in permanently shadowed regions in craters near the poles. 37 Measure the albedo (at Laser wavelength of 1064 nm) passively (without Laser 38 7 pulse) on the Sun-illuminated surface 39 Measure surface roughness globally and locally by analysing the shape of the return 40 8 41 pulse 42 43 1.2 Properties of the return pulse 44 45 Laser altimetry can provide measurements of the structure and albedo of the target surface in addition to the straightforward 46 range measurement. If p(t) is the temporal dependence of the received number of photons in the return pulse, the three 47 main parameters which can be extracted can be described as [2] 48 ∞ 49 푁 = ∫ 푝(푡) 푑푡 (1) 50 0 51 52 where N is the number of photons in the return pulse (a quantity related to the surface albedo). 53 54 55 56 57 58 59 60 61 62 63 64 65 1 ∞ 푇 = ∫ 푡 푝(푡)푑푡 (2) 1 푝 푁 2 0 3 4 where Tp is the pulse centroid time (a quantity related to the surface range) and 5 ∞ 2 1 2 6 휎푝 = ∫ (푡 − 푇푝) 푝(푡) 푑푡 (3) 7 푁 0 8 9 where σp, is the rms pulse width (a quantity related to the surface roughness and slope). The BELA instrument is designed 10 to obtain the range to the surface to high accuracy but also to place constraints on the surface albedo (particularly in 11 permanently unilluminated crater bottoms at Mercury’s poles) and the small scale (below 30 m) surface roughness. 12 13 14 15 2. INSTRUMENT DESCRIPTION 16 17 2.1 Link budget 18 BELA was separated into two major sub-systems to allow stand-alone testing in the two main contributing countries, 19 Switzerland and Germany. The transmitter section of the instrument was under the responsibility of DLR and comprised 20 the laser, its associated electronics and baffling, and the system level electronics box (incorporating the Spanish provided 21 power converter). The receiver section of the instrument was under the responsibility of the University of Bern and 22 comprised the receiving optics and associated baffling, the detector and the range-finding electronics. System integration 23 was performed at the University of Bern. 24 25 The link budget scales the laser pulse energy and the receiver aperture area. The return pulse energy at nadir can be 26 computed using the link equation 27 푆 퐴 28 푁 퐸푟 = 퐸푟푇푟 (4) 29 푧2 휋 30 where Er is the received pulse energy, Et is the emitted pulse energy, Tr is the receiver optics transmission, S is the collecting 31 area of the telescope, AN is the target surface reflectivity and z is the altitude of the spacecraft above the surface [3,4]. The -1 32 quantity AN/π is sometimes known as the target backscattering (coefficient) and has units of [sr ] [5]. A detailed trade-off 33 was needed to evaluate the best configuration taking into account the size of the aperture that BELA would need to have 34 in the thermal shield of the spacecraft. The trade-off resulted in specification of a Nd:YAG laser emitting at 1064 nm 35 (frequency-doubling was found to be less efficient partially because of the redness of Mercury itself) operating with a 36 nominal pulse energy of 50 mJ at 10 Hz with a 5 ns pulse duration.
Details
-
File Typepdf
-
Upload Time-
-
Content LanguagesEnglish
-
Upload UserAnonymous/Not logged-in
-
File Pages12 Page
-
File Size-