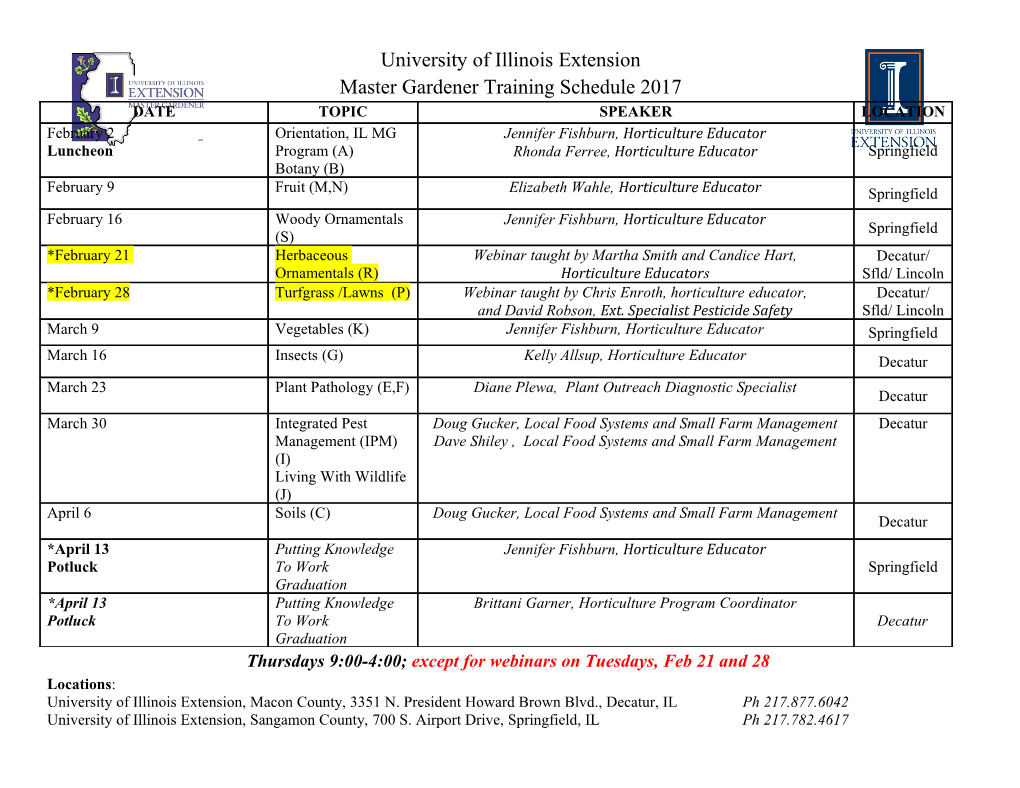
FINAL REPORT Development and Optimization of the Direct Carbon Fuel Cell Professor Scott Donne University of Newcastle February 26, 2016 Final Report, 2016 Submitted to Coal Innovation NSW Project Commence Date: April, 2011 Project Cessation Date: December 2015 Project Duration: 5 years Funding Allocated: $564,748 Phone: (02) 4921 5477 Fax: (02) 4921 5472 Email: [email protected] Address: Discipline of Chemistry, University Drive, Callaghan NSW 2308, Australia 1 Executive Summary Professor Scott Donne and co-investigators at the University of Newcastle (UoN) are investigating the Direct Carbon Fuel Cell (DCFC), a technology that has the ability to efficiently utilize coal to produce electricity with minimal environmental greenhouse gas emissions. The DCFC is not a new technology, having been first demonstrated by Grove in 1839, and then investigated further by Jacques in 1896 (US Patent 555,511) for the direct production of electricity. While this system demonstrated the concept, it was expensive at the time and encountered problems with system impurities. Since then a number of researchers have occasionally revisited the DCFC, gradually making fundamental and performance improvements, until the present time when the DCFC is now viewed as a viable alternative for coal-fired energy generation. In fact, the basic chemical reaction used by a coal fired power station and a DCFC are the same. The only difference is that the DCFC directly converts solid carbon into electrical energy through an electrochemical process where oxygen is combined with the carbon, as shown in the following schematic. - Here the cathodic process is the reduction of oxygen (O2) in air with electrons (e ) from the external circuit to form oxide anions (O2-). These oxide anions then pass through a solid oxide ion conductor (electrolyte) to be combined with carbon dioxide (CO2) to make carbonate 2- ions (CO3 ). These carbonate ions act a carriers for the oxide anions to ultimately be combined with the carbon fuel via oxidation to make carbon dioxide and the electrons that pass through the external circuit. The potential difference between the anode and cathode in the DCFC produces a cell voltage, in a similar way that a battery works. Current commercial systems operating in NSW generate electricity operate at most ~45% thermal efficiency and produce carbon dioxide (CO2) that has to be subsequently captured and purified before storage (carbon sequestration). In contrast, the DCFC system is expected to operate at >80% thermal efficiency and produce pure CO2. The CO2 generated is approximately half the volume of that produced by current systems as well, which further diminishes greenhouse gas emissions. This technology will enable rapidly declining coal reserves to be used in a highly efficient way. The DCFC will also minimize or remove associated by-process costs involved in capturing 2 the CO2 (called post-combustion capture, PCC) and purifying the CO2 prior to carbon sequestration. This impact of this novel DCFC technology research has the potential to be world- changing and hold considerable significant commercialization advantages for the NSW Coal Industry. In summary, the DCFC offers the Australian and international coal industries: (i) A way to extend their longevity (profitability and sustainability) (ii) An effective tool to responsibly meet the ever increasing demands for electricity (social impact) (iii) Reduce the carbon footprint imposed by the industry (environmental and social impact). The overall objective of this project is to obtain proof of concept (POC) for the technology and progress towards commercialisation of the DCFC through further development and optimisation of bench-scale and then pilot-scale systems. Coal Innovation NSW began funding Prof. Scott Donne at UoN in April 2011. This project revolves around the development and optimization of the Direct Carbon Fuel Cell (DCFC). The project objectives include: (i) To understanding the mechanism through which coal is oxidized to form CO2, and identifying and optimizing the important process variables for optimum performance, as defined by the kinetics of carbon oxidation to CO2. The important process variables include the type and impurity level of the coal and whether coal washing is necessary, the nature and composition of the electrolyte (molten carbonate), the anodic catalyst, the operational temperature, and the gas-phase composition (CO2/O2 ratio). (ii) Development of an operational (bench and pilot scale) DCFC, in particular, taking into account the mode of coal introduction to the catalyst surface. The original concept of a fuel cell was first demonstrated by Grove in 1839. Although development of the DCFC has been underway since 1896, it is still in early developmental stages due to the substantial technical challenges that remain that require further research and development. This includes complex issues of materials degradation, reaction kinetics, stack fabrication, fuel delivery and system design. The identified critical success factors for this project will be: (i) Optimising performance of the coal oxidation process. (ii) Overcoming limitations of the type of coal that can be used and preparation steps required. (iii) Development of an operational bench and pilot scale DCFC (proof of concept). A number of commercial partners have been approached during the course of this project, particularly in China where the market for small to medium size energy generation is quite large. Fundamental investigations of the electrochemical oxidation if carbon carried out in this project have shown several avenues for improving performance of a pilot scale DCFC. These findings have allowed focus on optimisation work of the solid carbon anode to be planned including pre-treatment of the raw coal material using several different methods. Methods thought to increase electrochemical activity include pyrolysis and partial oxidation as well as the discovery of a high impact solid catalyst (kaolin) which enables dramatic enhancement of the oxidation kinetics. This catalyst is both cheap and abundant and easily incorporated into the solid 3 coal electrode through physical mixing. Review of reported DCFC arrangements and performances has also allowed us to pin-point key areas which will allow a highly optimised and high performing pilot scale cell to be developed. In particular, it has been found that use of a solid anode in molten carbonate is the most advantageous arrangement and is expected to improve on reported performances. The final design of the pilot scale DCFC is thought to be superior to other designs as it is the only arrangement using a solid anode as well as the only arrangement to allow flexibility and optimisation in terms of the solid oxide electrolyte and cathode catalyst. The arrangement also allows off-gas sampling and analysis and careful control and monitoring of operational parameters such as temperature, pressure and purge gas composition. Through application of findings from previously investigated milestones (including mechanistic investigations, catalyst development, coal type and pre-treatment effect, molten carbonate composition and contamination effects) and realisation of current cell design a highly optimised pilot-scale DCFC is expected to be realised on project completion. Key scientific and technological outcomes from this project, compiled into their respective research areas, include: (i) Electrolyte Composition: Common coal impurities were all found to affect the melt temperature of the electrolyte, either lowering or raising the melting point, indicating dissolution of the contaminant. Dissolution of these coal impurities had minimal impact on coal oxidation kinetics. This dissolution of impurities has also been discussed in terms of an opportunity to reclaim value added materials from the electrolyte component. (ii) Carbon Properties: A range of different coal types (bother thermal and metallurgical) and their pre- pyrolysis has been investigated for their effect on coal oxidation kinetics. All coal samples behaved better than the baseline graphite because of their lower stability. It was found that thermal pre- treatment activated the coals via removing volatiles and functionality, and increased graphitisation. Lower pre-treatment temperatures removed volatiles but did not enhance coal conductivity to achieve good electrode performance. Higher pre-treatment temperatures led to significant loss of functionality, together with coal graphitisation. An optimum pre- treatment temperature was found depending on the coal type, established as a trade-off between functionalization and graphitization (conductivity). (iii) Catalysis: It was found that most common impurities in coal improve anode performance, and in the worst case, there was no effect on performance. A clay catalyst (kaolin) had a profound effect on performance; e.g., 5 wt% kaolin in coal doubled anodic current output. The distribution of kaolin in the anode was important, suggesting a surface mediated oxide ion transfer process. This is a new finding. (iv) Carbon Oxidation Mechanism: A thorough electrochemical kinetic analysis of the carbon oxidation mechanism was carried out, assuming that each step in the mechanism was the rate determining step for electrochemical oxidation. Application of the predicted kinetic parameters to electrochemical data enabled identification of the rate determining step as a function of active material type and condition, as well as applied overpotential. In
Details
-
File Typepdf
-
Upload Time-
-
Content LanguagesEnglish
-
Upload UserAnonymous/Not logged-in
-
File Pages177 Page
-
File Size-