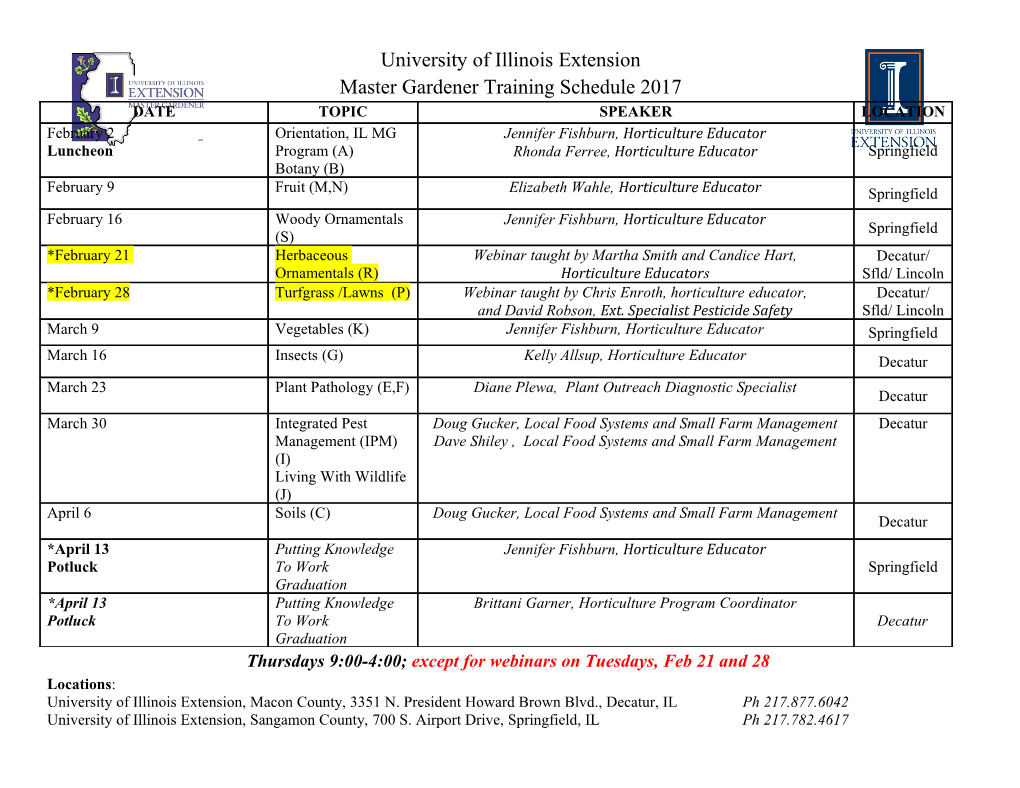
MHF Preprint Series Kyushu University 21st Century COE Program Development of Dynamic Mathematics with High Functionality The Littlewood-Paley-Stein inequality for diffusion processes on general metric spaces H. Kawabi & T. Miyokawa MHF 2006-27 ( Received September 11, 2006 ) Faculty of Mathematics Kyushu University Fukuoka, JAPAN The Littlewood-Paley-Stein inequality for diffusion processes on general metric spaces Hiroshi KAWABI Faculty of Mathematics Kyushu University 6-10-1, Hakozaki, Higashi-ku, Fukuoka 812-8581, JAPAN e-mail: [email protected] and Tomohiro MIYOKAWA Department of Mathematics, Graduate School of Science Kyoto University Sakyo-ku, Kyoto 606-8502, JAPAN e-mail: [email protected] Abstract: In this paper, we establish the Littlewood-Paley-Stein inequality on general metric spaces. We show this inequality under a weaker condition than the lower bounded- ness of Bakry-Emery’s Γ2. We also discuss Riesz transforms. As examples, we deal with diffusion processes on a path space associated with stochastic partial differential equations (SPDEs in short) and a class of superprocesses with immigration. Mathematics Subject Classifications (2000): 42B25, 60J60, 60H15. Keywords: Littlewood-Paley-Stein inequality, gradient estimate condition, Riesz trans- forms, SPDEs, superprocesses. 1 Framework and Results In this paper, we discuss the Littlewood-Paley-Stein inequality. After the Meyer’s cele- brated work [16], many authors studied this inequality by a probabilistic approach. Espe- cially, Shigekawa-Yoshida [20] studied to symmetric diffusion processes on a general state space. In [20], they assumed that Bakry-Emery’s Γ2 is bounded from below. To define Γ2, they also assumed the existence of a suitable core A which is not only a ring but also stable under the operation of the semigroup and the infinitesimal generator. However, 1 in general, it is very difficult to check the existence of A having good properties de- noted above. Hence their assumption is serious when we face several infinite dimensional diffusion processes. In this paper, we show that the Littlewood-Paley-Stein inequality also holds on gen- eral metric spaces under the gradient estimate condition (G) even if we do not assume the existence such a core A. Our condition seems somewhat weaker than the lower boundedness of Γ2. We mention that Coulhon-Duong [5] and Li [14] also discussed the Littlewood-Paley-Stein inequality under similar conditions on finite dimensional Rieman- nian manifolds. Contrary to these papers, we work on a more general framework to handle certain infinite dimensional diffusion processes in Section 4. We introduce the framework that we work in this paper. Let X be a complete sep- arable metric space. Suppose we are given a Borel probability measure ¹ on X and a local ¹-symmetric quasi-regular Dirichlet form E in L2(¹) with the domain D(E). See Ma-R¨ockner [15] for the terminologies of quasi-regular Dirichlet forms. Then by Theorem 1.1 of Chapter V in [15], there exists a ¹-symmetric diffusion process M := (Xt; fPxgx2X ) associated with (E; D(E)). We denote the infinitesimal generator and the transition semi- group by L and fPtgt¸0, respectively. Since fPtgt¸0 is ¹-symmetric, it can be extended p to the semigroup on L (¹); p ¸ 1. We denote it by fPtgt¸0 again. We also denote its p generator in L (¹) by Lp and the domain by Dom(Lp), respectively if we have to specify the acting space. We assume that 1 2 Dom(Lp) and Lp1 = 0 for all p ¸ 1, where 1 denotes the function that is identically equal to 1. In particular, the diffusion process M is conservative. Throughout this paper, we impose the following condition: (A): There exists a subspace A of Dom(L2) consisting of bounded continuous function 2 which is dense in D(E) and f 2 Dom(L1) holds for any f 2 A. Under this condition, the form E admits a carr´edu champ, namely, there exists a unique positive symmetric and continuous bilinear form Γ from D(E)£D(E) into L1(¹) such that Z E(fh; g) + E(gh; f) ¡E(h; fg) = 2 hΓ(f; g) d¹ X 1 holds for any f; g; h 2 D(E) \ L (¹). In particular, for f; g 2 Dom(L2), fg 2 Dom(L1) and 1© ª Γ(f; g) = L (fg) ¡ (L f)g ¡ f(L g) 2 1 2 2 hold. For further information, see Theorem 4.2.2 of Chapter I in Bouleau-Hirsch [4]. In the sequel, we also use the notation Γ(f) := Γ(f; f) for the simplicity. The following gradient estimate condition is crucial in this paper. (G): There exist constants K > 0 and R 2 R such that the following inequality holds for any f 2 A and t ¸ 0: 2Rt © ª Γ(Ptf) · Ke Pt Γ(f) : (1.1) 2 Remark 1.1 If we can see A is stable under the operations of fPtg and L, Γ2(f) ¸ ¡RΓ(f); f 2 A (1.2) ¡ ¢ 1 implies (1.1) with K = 1, where Γ2(f) := 2 L1Γ(f) ¡ 2Γ(L2f; f) : Especially, (1.2) means that the Ricci curvature is bounded by ¡R from below in the case where X is a finite dimensional complete Riemannian manifold. See Proposition 2.3 in Bakry [2] for details. Hence our condition (G) is weaker than (1.2). Let us introduce the Littlewood-Paley G-functions. To do this, we recall the sub- ordination of a semigroup. For t ¸ 0, we define a probability measure ¸t on [0; +1) by t 2 ¸ (ds) := p e¡t =4ss¡3=2ds: t 2 ¼ In terms of the Laplace transform, this measure is characterized as Z 1 p ¡γs ¡ γt e ¸t(ds) = e ; γ > 0: 0 (®) For ® ¸ 0, we define the subordination fQt gt¸0 of fPtgt¸0 by Z 1 (®) ¡®s p Qt f := e Psf ¸t(ds); f 2 L (¹): 0 Then we can easily see that Z 1 (®) ¡®s kQt fkLp(¹) · e kPsfkLp(¹)¸t(ds) 0Z ³ 1 ´ p ¡®s ¡ ®t · e ¸t(ds) kfkLp(¹) = e kfkLp(¹); (1.3) 0 and hence fQ(®)g is a strongly continuous contraction semigroup on Lp(¹). The in- t t¸0 p (®) 2 p finitesimal generator of fQt pgt¸0 in L (¹) is ¡ ® ¡ L. In the case of L (¹), this operator will be clearly denoted by ¡ ® ¡ Lp when the dependence of p is significant. For f 2 L2 \ Lp(¹) and ® > 0, we define Littlewood-Paley’s G-functions by ¯ ¯ µZ ¶ ¯ ¯ 1 1=2 ! ¯ @ (®) ¯ ! ! 2 gf (x; t) := ¯ (Qt f)(x)¯ ;Gf (x) := tgf (x; t) dt ; @t 0 µZ 1 ¶1=2 " ¡ (®) ¢1=2 " " 2 gf (x; t) := Γ(Qt f) (x);Gf (x) := tgf (x; t) dt ; 0 q µZ 1 ¶1=2 ! 2 " 2 2 gf (x; t) := (gf (x; t)) + (gf (x; t)) ;Gf (x) := tgf (x; t) dt : 0 Now we present the Littlewood-Paley-Stein inequality. In what follows, the notation kukLp(¹) . kvkLp(¹) stands for kukLp(¹) · CkvkLp(¹), where C is a positive constant depending only on K and p. 3 Theorem 1.2 For any 1 < p < 1 and ® > R _ 0, the following inequalities hold for f 2 L2 \ Lp(¹): kGf kLp(¹) . kfkLp(¹); (1.4) ! kfkLp(¹) . kGf kLp(¹): (1.5) Before closing this section, we give an application of Theorem 1.2. It plays an impor- tant role in the regularity theory of parabolic PDEs on general metric spaces. Theorem 1.3 Let 1 < p < 1; q ¸ 1 and ® > R _ 0. We define p (q) ¡ ¡q ¢1=2 p R® (L)f := Γ ( ® ¡ Lp) f ; f 2 L (¹): Then we have the following statements: (q) p (1) For any p ¸ 2 and q > 1, R® (L) is bounded on L (¹). Moreover there exists a (q) positive constant kR® (L)kp;p depending only on K; p; q and ®R := (® ¡ R) ^ ® such that ° ° (q) (q) p ° ° p R® (L)f Lp(¹) · kR® (L)kp;pkfkL (¹); f 2 L (¹): (1.6) This implies the inclusion p ¡ q¢ 1;p © p 1=2 p ª Dom ( 1 ¡ Lp) ½ W (¹) := f 2 L (¹) \D(E) j Γ(f) 2 L (¹) : (2) For any p ¸ 2 and 1 < q < 2, there exists a positive constant Cp;q such that ° ° 1=2 (q) ¡ q=2 ¡q=2¢ p ° ° p Γ(Ptf) Lp(¹) · Cp;qkR® kp;p ® + t kfkL (¹); t > 0; f 2 L (¹): (1.7) Remark 1.4 We do not know whether our gradient estimate condition (G) is sufficient or not to establish (1.6) for q = 1, i.e., so-called the boundedness of the Riesz trans- (1) p form R®(L) := R® (L) on L (¹), Recently, Shigekawa [18] discussed the boundedness of R®(L) under the intertwining condition for the diffusion semigroup in a general frame- work. We remark that the intertwining condition implies (G). Hence one way to establish the boundedness of R®(L) is to show the intertwining condition for each concrete problem. 2 Proof of Theorem 1.2 In this section, we prove Theorem 1.2 by a probabilistic method. The original idea is due to Meyer [16]. The reader is referred to see also Bakry [1], Shigekawa-Yoshida [20] and (®) p Yoshida [24]. In these papers, they expanded L(Qt f) ; f 2 A, by employing the usual functional analytic approach for the proof of the Littlewood-Paley-Stein inequality. Note that this approach is valid because they imposed the existence of a good core A described in Section 1. On the other hand, in this paper, A in condition (A) does not have such good properties. So we cannot draw their proof directly. To overcome this difficulty, 4 we need more delicate probabilistic arguments based on Itˆo’sformula.
Details
-
File Typepdf
-
Upload Time-
-
Content LanguagesEnglish
-
Upload UserAnonymous/Not logged-in
-
File Pages29 Page
-
File Size-