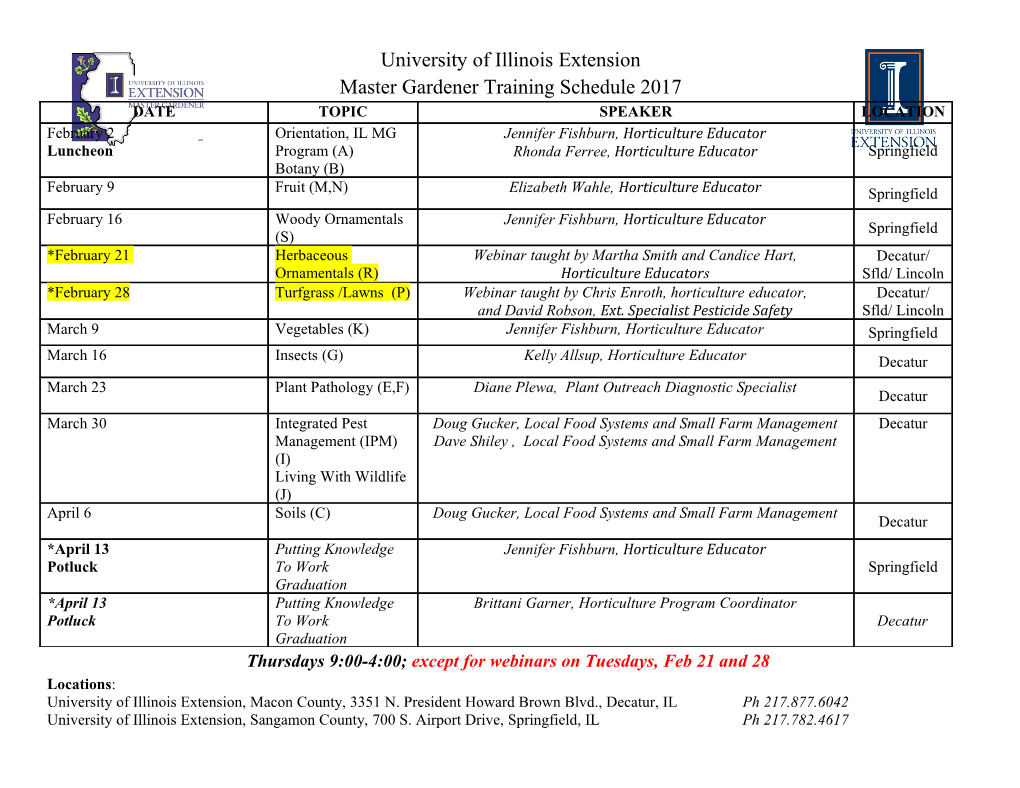
Planetary Science Vision 2050 Workshop 2017 (LPI Contrib. No. 1989) 8056.pdf E-SAIL FOR FAST INTERPLANETARY TRAVEL. M. Aru1, P. Janhunen2, 1University of Tartu, Estonia, 2Finnish Meteorological Institute, Finland Introduction: Propulsion is a significant factor for areas of scientific research and new types of missions our access to the Solar System and the time con- could be imagined and created, improving our unders- sumption of the missions. We propose to use the elect- tanding of the Solar System. ric solar wind sail (E-sail), which can provide remar- Manned presence on Mars. A spacecraft equipped kable low thrust propulsion without needing propellant with a large E-sail, that provides 1 N of thrust at 1 au [1, 2]. from Sun, can travel from Earth to the asteroid belt in a The E-sail is a propellantless propulsion concept year. One such spacecraft can bring back three tonnes that uses centrifugally stretched, charged tethers to of water in three years, and repeat the journey multiple extract momentum from the solar wind to produce times within its estimated lifetime of at least ten years thrust. Over periods of months, this small but conti- [9, 12]. The water can be converted to synthetic cryo- nuous thrust can accelerate the spacecraft to great genic rocket fuel in orbital fuelling stations where speeds of approximately 20 to 30 au/year. For examp- manned vehicles travelling between Earth and Mars le, distances of 100 au could be reached in <10 years, can be fuelled. This dramatically reduces the overall which is groundbreaking [1]. mission fuel ratio at launch, and opens up possibilities The principles of operation: A full-scale E-sail for affordable continuous manned presence on Mars includes up to 100 thin, many kilometers long tethers, [13]. which are held at a high positive potential by an Multi-asteroid touring. Asteroids are of significant onboard electron gun. A high-voltage charge on the interest since they not only contain valuable resources tethers deflects the solar wind protons that flow ra- and pose a danger of impacting the Earth, but also pro- dially away from the sun which results in a reaction vide insights into the origin of the Solar System. A force on the tethers. The thrust vector, which points proposal to compose a survey of hundreds of asteroids roughly radially away from the sun, can be turned wit- by a fleet of nanosatellites was submitted to the Euro- hin a ~30° cone by inclining the sail [3]. The sail’s pean Space Agency’s “Call for new ideas” call in Sep- thrust is proportional to the product of the solar wind tember, 2016. Each CubeSat carries a single tether as a dynamic pressure and the effective sail area. The range downscaled E-sail and is equipped with a lightweight of the tethers’ electric field can be about a million ti- optical and near infrared imaging system. Image data mes larger than the tether itself, which more than allows us to measure the asteroid’s albedo and size compensates for the very weak dynamic pressure of the during a flyby, while spectral data allows us to detect solar wind [4]. surface minerals of the studied asteroids. Furthermore, An E-sail’s operating principle differs from the so- information about surface geology, geophysics, and lar sail, which is based on momentum transfer from thermal properties can be obtained. Knowing solar photons [5]. The thrust produced by an E-sail geophysical properties of asteroids such as mass, inte- decreases as 1/r (where r is the solar distance) and it rior structure and composition is needed for selecting provides acceleration to distances up to 30 au. In suitable targets and technologies for asteroid mining, comparison, a solar sail’s thrust declines at a rate of and assessment of the asteroid impact threat. Further- 1/r2 and is capable of accelerating a spacecraft to only more, a statistical view to size and compositional dist- ~5 au [6]. ributions for asteroid families is important for constrai- Applications: The E-sail’s acceleration is up to 2 ning the Solar System’s evolution models. Asteroid mm/s2 in maximum, but over time great speeds would families are vital for studying the composition and be achieved. Missions to Saturn and Jupiter can be structure of the planetesimals from which Earth and accomplished in 1-2 years. Neptune and Uranus can be other planets once formed [14]. reached in 3-5 years. Furthermore, an E-sail mission to The size of the fleet is scalable. Each spacecraft the Heliopause would take 10 to 15 years, while Voya- makes a flyby of 6-7 asteroids, typically, and the fleet ger spacecrafts travelled there for 36 years. of 50 will study a groundbreaking number of 300+ Since the sail can produce continuous thrust, non- near-Earth objects and mainbelt asteroids. They could Keplerian orbits and stable off-Lagrange positions be launched by India’s Polar Satellite Launch Vehicle could be maintained [7]. Other applications include (PSLV), for example. inner planets and sample return missions, asteroid def- Opportunities for planetary science. The E-sail can lection, multi-asteroid touring, and flyby or orbiter be used for flyby missions towards inner and outer pla- missions to outer planets [4, 8, 9, 10, 11]. Thus, new nets of the Solar System. Examples include remote Planetary Science Vision 2050 Workshop 2017 (LPI Contrib. No. 1989) 8056.pdf sensing of planets from non-Keplerian orbits, gas giant Mengali. (2010) Acta Astronaut., 66, 1506–1519. [10] planet atmosphere probe, and deep space planetary or A. Quarta and G. Mengali. (2010) J. Guid. Contr. planetary moon flyby [15]. Dyn., 33, 740–755. [11] A. Quarta, G. Mengali, P. A sample return mission is possible as well, as it Janhunen. (2011) Acta Astronaut., 68, 603–621. [12] has been analyzed for an asteroid. The mission can be A. Quarta, G. Mengali, P. Janhunen. (2014) Journal of divided into three phases. In the first phase, the E-sail Aerospace Engineering 27 04014031. based spacecraft travels from the Earth’s heliocentric doi:10.1061/(ASCE)AS.1943-5525.0000285. [13] P. orbit to the target’s orbit, and maintains a determined Janhunen, S. Merikallio, M. Paton. (2015) Acta Astro- orbit relative to it after the rendezvous. In the second naut., 113, 22-28. [14] Masiero, F.E. DeMeo, T. Kasu- phase, a lander can be used to reach the object’s surfa- ga, and A.H. Parker. (2015) P. Michel et al. eds., 323– ce and collect material samples. At the end of that pha- 340, Univ. of Arizona, Tucson. [15] P. Janhunen et al. se, the lander performs a docking maneuver with the (2014) Planet. Space Sci., 104A, 141-146. [16] (2016, spacecraft. The third phase involves the return, and December 5). Retrieved from http://www.electric- ends with an Earth’s rendezvous [12]. sailing.com/projects.html E-sail projects [16]: ESAIL EU FP7 project (2010-2013): An interna- tional project during which laboratory prototypes of the electric sail’s key components were built. ESTCube-1 (2013-2015): The first satellite to test the working principles of E-sail in low Earth orbit. An attempt was made to deploy a 10 m long tether from the one-unit CubeSat by centrifugal force. However, the deployment was unsuccessful. NASA HERTS (Heliopause Electrostatic Rapid Transit System): A project for the development of E- sail at NASA's Marshall Space Flight Center. In April 2016, tests for examining the rate of proton and elect- ron collisions with a positively charged wire began at the High Intensity Solar Environment Test system. Aalto-1 (2017): A Finnish mission of a three-unit CubeSat, which will test a 100 m long E-sail tether for deorbiting the satellite in Earth’s magnetosphere. This is planned for the end of the satellite’s operational li- fespan to avoid the creation of space debris. ESA Asteroid Touring by Electric Sail Technology study, 2015-2017. ESTCube-2 (planned for 2018): An Estonian mis- sion of a three-unit CubeSat, which will test a 300 m long E-sail tether in a similar way to Aalto-1. ESTCube-3: An Estonian mission of a three-unit CubeSat with a goal to orbit the Moon, which the solar wind reaches unlike the Earth’s magnetosphere. References: [1] B. Wiegmann. (2014) NASA MSFC. [2] P. Janhunen. (2004) J. Prop. Power, 20, 763-764. [3] P. Toivanen, P. Janhunen. (2013) J. Prop. Power 29, 178–185. doi:10.2514/1.B34330. [4] G. Mengali, A. Quarta, P. Janhunen. (2008) J. Spacecr. Rockets, 45, 122-129. [5] C. R. McInnes. (2004) Springer. [6] P. Janhunen, et al. (2010) Rev. Sci. Instr. 81, 111301. [7] G. Mengali and A. Quarta. (2009) Cel. Mech. Dyn. Astron., 105, 179-195, doi:10.1007/s10569-009-9200-y. [8] S. Merikallio, P. Janhunen. (2010) Astrophys. Space Sci. Trans., 6, 41– 48. doi:10.5194/astra-6-41-2010. [9] A. Quarta and G. .
Details
-
File Typepdf
-
Upload Time-
-
Content LanguagesEnglish
-
Upload UserAnonymous/Not logged-in
-
File Pages2 Page
-
File Size-