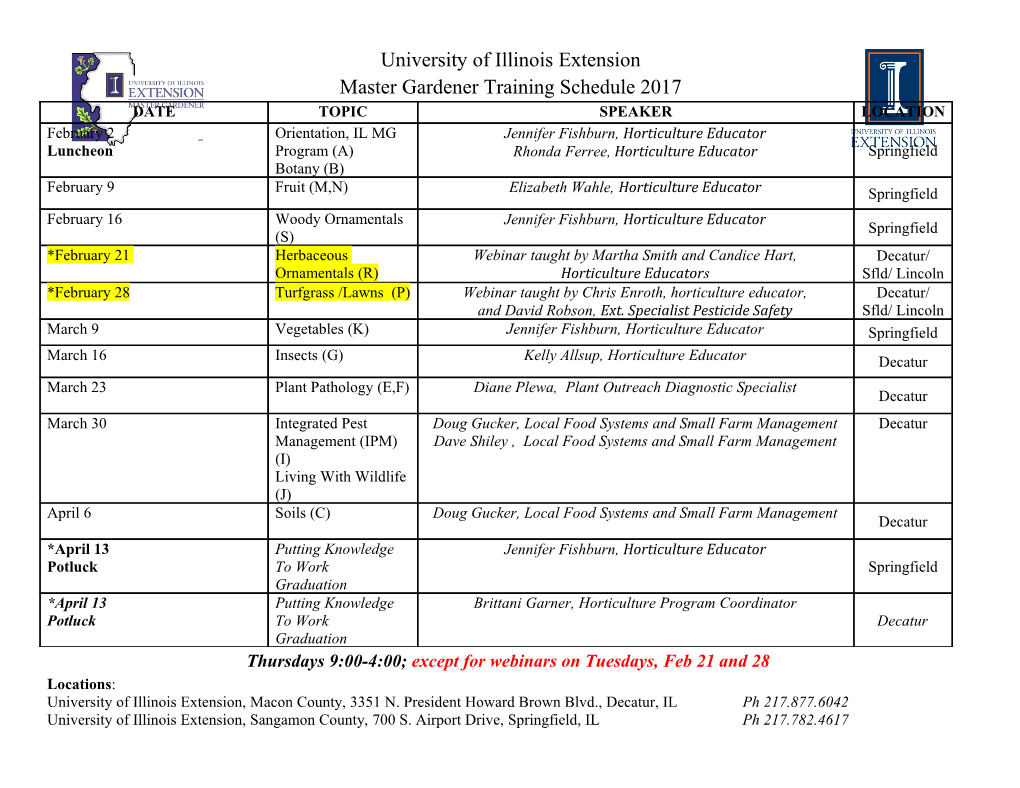
Earth’s Outgassing and Climatic Transitions: The Slow Burn Towards Environmental “Catastrophes”? N. Ryan McKenzie1 and Hehe Jiang2 1811-5209/19/0015-0325$2.50 DOI: 10.2138/gselements.15.5.325 n multimillion-year timescales, outgassing from the Earth’s interior Solid Earth carbon degassing occurs at mid-ocean ridges, rifts, provides the principal source of CO2 to the ocean–atmosphere system, volcanic arcs in subduction zones, Owhich plays a fundamental role in shaping the Earth’s baseline climate. orogenic belts, and large igneous Fluctuations in global outgassing have been linked to icehouse–greenhouse provinces (FIG. 1). The global transitions, although uncertainties surround paleo-outgassing fluxes. Here, we volcanic flux of CO2 probably varied significantly throughout discuss how volcanic outgassing and the carbon cycle have evolved in concert geologic time. Changes in subduc- with changes in plate tectonics and biotic evolution. We describe hypotheses tion zone length, in the volume of driving mechanisms for the Paleozoic icehouse–greenhouse climates and of a mantle plume, and/or in the explore how climatic transitions may have influenced past biotic crises and, global oceanic crust production rate can result in at least a two-fold in particular, how variable outgassing rates established the backdrop for increase in volcanic CO2 inputs carbon cycle perturbations to instigate prominent mass extinction events. (Lee et al. 2013). Variation in the CO2 input would, therefore, play KEYWORDS: carbon cycle, outgassing, weathering, climate, biodiversity, a fundamental role in changing mass extinction long-term baseline climate states. These tectonic/magmatic processes LONG-TERM CARBON CYCLE also contribute to modifying the Earth has transitioned between two major baseline climate climate–silicate weathering feedback strength, which is states throughout its history: icehouse (or Ice Age) intervals influenced by the exhumed supply of weatherable minerals, that consist of generally cool climates with well-developed dissolution kinetics, and hydrologic conditions (Hartmann polar ice sheets, and warm greenhouse intervals devoid et al. 2014; Maher and Chamberlain 2014; Jiang and Lee of ice caps. Transitions between icehouse and greenhouse 2019). The long-term carbon cycle stabilizes planetary intervals are largely influenced by the partial pressure climate due to the temperature sensitivity of silicate weath- of atmospheric carbon dioxide (pCO2). The transfer of ering—at high temperatures weathering rates increase and carbon between the solid Earth (endogenic system) and rapidly draw CO2 out of the atmosphere, so cooling the the ocean–atmosphere (exogenic system) on million-year climate. Because surface temperature is, in part, controlled timescales is governed by the long-term carbon cycle. The by pCO2, the relation between CO2 inputs and the weath- pCO2 is a result of the balance between the rate of CO2 ering feedback can be represented as the curve in FIGURE 2. inputs through magmatic/metamorphic degassing and the In general, we expect a positive correlation between the rates of carbon removal via silicate weathering and organic input or output with steady state pCO2, with the slope of carbon burial (Berner 2004) (FIG. 1). Due to the small size the curve representing the feedback strength. With linear of the surface carbon reservoir relative to the inputs from the solid Earth, a negative feedback silicate weathering between silicate weathering carbonate burial carbonate weathering Volcanic, metamorphic and temperature is required to Corg burial Corg weathering CO2 degassing stabilize atmospheric CO2 and climate on timescales of longer than 105 years (Walker et al. 1981). Thus, steady-state pCO2 levels are determined by the CO degassing input and the relative strength of 2 the silicate weathering feedback MOR mechanisms (Berner 2004). 1 Department of Earth Sciences Schematic of tectonic controls on the long-term FIGURE 1 University of Hong Kong carbon cycle. Oceanic crust is in light grey; Pokfulam, Hong Kong sediments on the oceanic crust and that form the accretionary E-mail: [email protected] prism are in light green; limestones (unmetamorphosed and metamorphosed) in blue; granite bodies in red. Abbreviations: 2 Department of Earth Sciences C = organic carbon; MOR = mid-ocean ridge. University of Toronto org Toronto, ON, Canada E-mail: [email protected] ELEMENTS, VOL. 15, PP. 325–330 325 OCTOBER 2019 kinetically supply ICEHOUSE–GREENHOUSE CONDITIONS stable climate limited limited IN DEEP TIME Since the Cryogenian Period (716–635 Ma), Earth has shifted between numerous icehouse–greenhouse states upper threshold (FIG. 3). The Cryogenian was one of the most extensive icehouses, being characterized by two Snowball Earth events when ice sheets reached paleo-shorelines in equato- rial regions. The first of these Snowball Earth events, input known as the Sturtian glaciation, occurred ~716 Ma, and or the second was the Marinoan glaciation that terminated ~635 Ma. Subsequent relatively short-lived, yet expansive, output glaciations included the Ediacaran Gaskiers glaciation (~580 Ma) and the Hirnantian glaciation at the end of the Ordovician Period (~440 Ma). The Late Paleozoic icehouse lower threshold extended from the Carboniferous to the late Permian, and our current Cenozoic icehouse climate was initiated runaway runaway icehouse pCO2 greenhouse Cz Mesozoic Paleozoic Neoproterozoic Schematic illustration of climate states having varying N Pg K J Tr P C D S O Є Ediac. Cryogenian FIGURE 2 weathering feedback and outgassing conditions. Dispersal Pangea Assembly Dispersal Rodina Continental Low outgassing rates combined with kinetically limited weathering Breakup Breakup Configuration regimes allows Earth to reach a state where extensive icehouse (or snowball) conditions can be achieved. Conversely, elevated outgas- Collision “Big 5” Mass Extinction Rate sing combined with supply-limited weathering (i.e., a negligible 2.0 silicate weathering feedback) can push Earth into a runaway green- Extinctions Alpine–Himalayan house. ADAPTED FROM LEE ET al. (2019). 1.0 weathering feedback, the system remains regulated and runaway processes cannot happen. However, global or regional weathering processes can be either “rate-limited”, 0.0 wherein an excess of weatherable minerals are available and Proportion Genera Proportion the weathering rate is limited by kinetic rate, or “supply- 0.6 limited”, which occurs when all weatherable substrates Extinction are exhausted and the weathering rate is limited by the 0.4 supply of fresh materials. As such, the negative feedback mechanism may not scale linearly with exogenic CO2 0.2 content. For example, in a low pCO2 scenario, tempera- ture could drop below some threshold where weathering kinetics become negligible because surface temperatures 0.0 are, in part, controlled by pCO2. As a result, the system p reaches a threshold of atmospheric pCO2 such that weath- 0.6 (hypercaliers) ering rates are insensitive to CO2, and further decreases in carbon input combined with ice albedo effects could 0.4 result in runaway icehouse conditions. Similarly, silicate weathering may become supply-limited even with high 0.2 “reef gap” “reef Cumulative Frequency Cumulative temperature and high pCO2, where the system reaches a Detrital Zircon Young 0.3 threshold above which increased CO2 does not result in increased weathering. This can result in runaway green- house conditions (FIG. 2). Therefore, “thresholds” in the silicate weathering feedback 0.2 exist, above or below which weathering rates become Increasing Young Young Increasing Zircon Proportion Proportion Zircon insensitive to pCO2, leading to failure of silicate weath- ering feedback in climate regulation (Foley 2015; Kump 2018). Crossing these thresholds would move the planet 0.1 to a new steady state with different feedback parameters. For example, in a low pCO2 scenario, temperature could drop below the water freezing point where weathering kinetics become sluggish, or even cease completely. If the Icehouse Icehouse Icehouse Cenozoic Cenozoic system maintains low CO2 input, the planet would remain Mesozoic- Greenhouse Greenhouse Cryogenian Gaskiers glaciation Sturtian glaciation Late Paleozoic Late Hirnantian glaciation Early Cenozoic Early Cenozoic Marinoan glaciation ice-covered and be dominated by runaway ice-albedo Early Paleozoic feedback. In a globally supply-limited scenario, the system 0 100 200 300 400 500 600 700 800 reaches a threshold above which increased CO2 does not Age (Ma) result in increased weathering, thus rapid emission of large Composite of climate states over last ~720 million quantity of CO2 may exhaust the capacity of the long- FIGURE 3 years, including the proportion of young detrital term climate regulator associated with silicate weathering, zircons, the proportion of benthic hypercalcifiers, the proportion of resulting in runaway greenhouse conditions (Foley 2015; genera extinctions, the extinction rates, and the various continental Kump 2018) (FIG. 2). configurations. Red = greenhouse conditions; blue = icehouse conditions. Ediac. = Ediacaran. MODIFIED AFTER MCKENZIE ET al. (2014, 2016), ALROY (2008), AND BAMbaCH ET al. (2004). ELEMENTS 326 OCTOBER 2019 during the later Paleogene (~33 Ma). Prominent green- assumed criteria for a prominent low-latitude weathering house intervals spanned the Ediacaran through the early system, yet the Himalaya modifies the climate system such Paleozoic, the middle Paleozoic, and the Mesozoic to early
Details
-
File Typepdf
-
Upload Time-
-
Content LanguagesEnglish
-
Upload UserAnonymous/Not logged-in
-
File Pages6 Page
-
File Size-