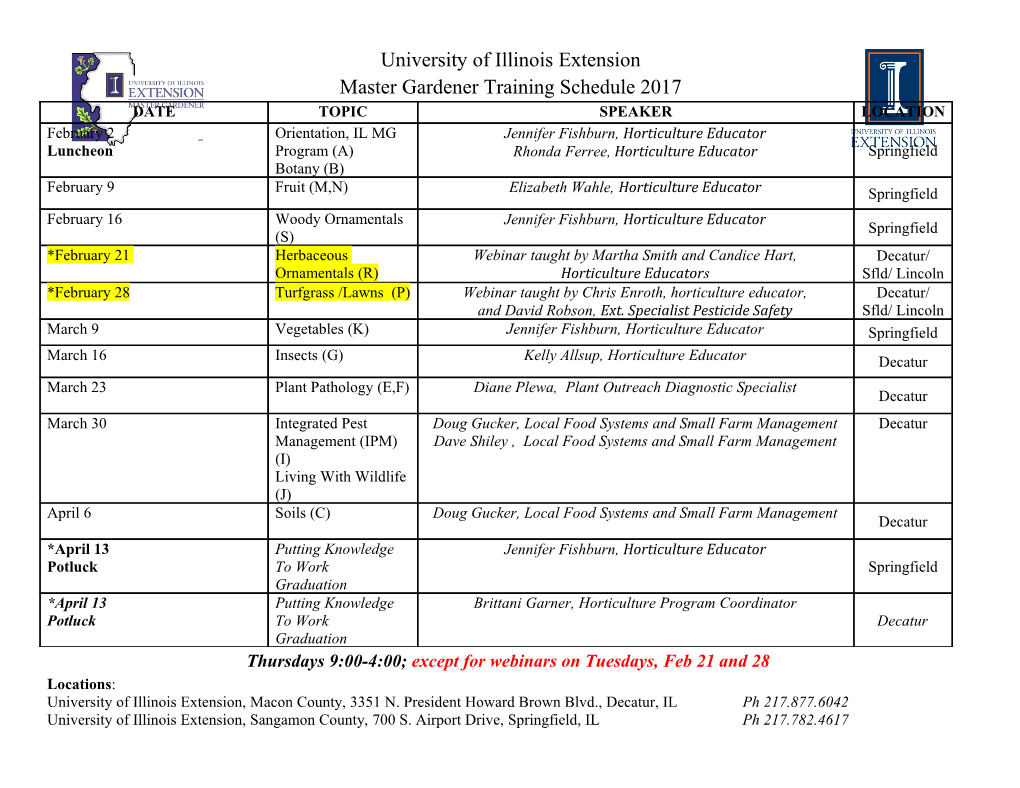
Biogeosciences, 15, 6867–6884, 2018 https://doi.org/10.5194/bg-15-6867-2018 © Author(s) 2018. This work is distributed under the Creative Commons Attribution 4.0 License. Riverine particulate C and N generated at the permafrost thaw front: case study of western Siberian rivers across a 1700 km latitudinal transect Ivan V. Krickov1, Artem G. Lim1, Rinat M. Manasypov1,2, Sergey V. Loiko1, Liudmila S. Shirokova2,3, Sergey N. Kirpotin1, Jan Karlsson4, and Oleg S. Pokrovsky3 1BIO-GEO-CLIM Laboratory, Tomsk State University, Lenina av., 36, Tomsk, Russia 2N. Laverov Federal Center for Integrated Arctic Research, Russian Academy of Sciences, 23 Naberezhnaya Severnoi Dviny, 163000, Arkhangelsk, Russia 3GET UMR 5563 CNRS, University of Toulouse, 14 Avenue Edouard Belin, 31400 Toulouse, France 4Climate Impacts Research Centre, Department of Ecology and Environmental Science, Umeå University, 901 87 Umeå, Sweden Correspondence: Oleg S. Pokrovsky ([email protected]) Received: 24 May 2018 – Discussion started: 25 June 2018 Revised: 12 October 2018 – Accepted: 29 October 2018 – Published: 19 November 2018 Abstract. In contrast to numerous studies on the dynamics of soils at the active latitudinal thawing front. The results sug- dissolved ( < 0:45 µm) elements in permafrost-affected high- gest that a northward shift of permafrost boundaries and an latitude rivers, very little is known of the behavior of river increase in active layer thickness may increase particulate C suspended (> 0:45 µm) matter (RSM) in these regions. In and N export by WSL rivers to the Arctic Ocean by a factor of order to test the effect of climate, permafrost and physio- 2, while P export may remain unchanged. In contrast, within geographical landscape parameters (bogs, forest and lake a long-term climate warming scenario, the disappearance of coverage of the watershed) on RSM and particulate C, N permafrost in the north, the drainage of lakes and transforma- and P concentrations in river water, we sampled 33 small tion of bogs to forest may decrease C and N concentrations and medium-sized rivers (10–100 000 km2 watershed) along in RSM by 2 to 3 times. a 1700 km N–S transect including both permafrost-affected and permafrost-free zones of the Western Siberian Lowland (WSL). The concentrations of C and N in RSM decreased with the increase in river watershed size, illustrating (i) the 1 Introduction importance of organic debris in small rivers which drain peat- lands and (ii) the role of mineral matter from bank abrasion High-latitude rivers are most vulnerable to ongoing climate in larger rivers. The presence of lakes in the watershed in- change via altering their hydrological regime (Bring et al., creased C and N but decreased P concentrations in the RSM. 2016) and widespread permafrost thaw that stimulates nutri- The C V N ratio in the RSM reflected the source from the deep ent release (Vonk et al., 2015). For carbon (C), the particulate soil horizon rather than surface soil horizon, similar to that of fraction (POC) contributes substantially to the total organic other Arctic rivers. This suggests the export of peat and min- C export from the continent to the ocean (Schlesinger and eral particles through suprapermafrost flow occurring at the Melack, 1981; Lal, 2003; Ludwig and Probst, 1996; Galy base of the active layer. There was a maximum of both partic- et al., 2015; Li et al., 2017; Coppola et al., 2018); a 2-fold ulate C and N concentrations and export fluxes at the begin- increase of Arctic rivers POC fluxes by 2100 is predicted ning of permafrost appearance, in the sporadic and discontin- (Gordeev and Kravchishina, 2009). Although the reasons for uous zone (62–64◦ N). This presumably reflected the organic strong variations of POC in freshwater are not yet fully un- matter mobilization from newly thawed organic horizons in derstood (Tian et al., 2015; Lee et al., 2016; Yang et al., Published by Copernicus Publications on behalf of the European Geosciences Union. 6868 I. V. Krickov et al.: Riverine particulate C and N across permafrost gradient 2016), the temperature (Hilton, 2017) and runoff (Goñi et al., concentrations in small rivers (Frey et al., 2007a; Frey and 2013) combined with local storm events (Jeong et al., 2012; McClelland, 2009). Although the majority of these features Wiegner et al., 2009) are widely recognized as the most im- were confirmed by a more recent study of dissolved carbon portant driving factors. This may be especially true for north- and nutrients in WSL rivers over main hydrological seasons ern aquatic systems, being highly sensitive to flood events, (Pokrovsky et al., 2015 and Vorobyev et al., 2017, respec- due to shallow water paths and a short transit time in water- tively), an assessment of particulate load transport in WSL sheds. rivers has not yet been performed, and the mechanisms con- Of special interest to POC of the Arctic rivers is that, if trolling particulate C, N and P mobilization from WSL soils soil organic C escapes degradation during river transport and to the Arctic Ocean remain unknown. is thus buried in marine sediments, it can contribute to a geo- To improve the current understanding of the magnitude logical carbon dioxide sink (e.g., Hilton et al., 2015). Further, and seasonality of riverine particulate nutrient exports, we the potentially increased transport of P and N may signif- quantified concentrations of C and macronutrients (N, P) icantly change primary productivity in riverine ecosystems across a vast latitudinal gradient (1700 km), with a special (Wrona et al., 2016; McClelland et al., 2007), thereby imped- emphasis on the permafrost-bearing zone during three main ing the rigorous predictions of climate change’s impact on hydrological regimes: (1) the peak of spring flood (early Arctic terrestrial–aquatic ecosystems. Despite significant ef- June 2016), (2) the summer base flow (August 2016) and forts in characterizing the fluxes, chemistry and origin of par- (3) the autumn high flow before the ice (October 2016). We ticulate organic matter (POM) in large Arctic rivers (Lobbes aimed to characterize the effect of latitude, permafrost cover- et al., 2000; Dittmar and Kattner, 2003; Unger et al., 2005; age and fundamental landscape features (proportion of bogs, Guo et al., 2004; Guo and Macdonald, 2006; Gladyshev et lakes and forest in the watershed) as well as the size of the al., 2015; Emmerton et al., 2008; McClelland et al., 2016; river itself on particulate C, N and P concentrations and the Gareis and Lesack, 2017), these studies do not allow for the relative fraction of particulate nutrient transport versus total assessment of mechanisms of POM generation in the water- (particulate C dissolved) nutrient transport. We further used shed. In particular, the role of the size of the river watershed acquired knowledge to infer the mechanisms of particulate and its landscape (physio-geographical) parameters are still nutrient mobilization from soils to rivers and applied these poorly known. Thus, although detailed studies of particulate mechanisms to predict change in particulate nutrient con- nutrients in small Arctic rivers helped to constrain seasonal centrations under climate warming, landscape evolution and features of export fluxes (Cai et al., 2008; Dornblaser and progressive permafrost thaw in the largest frozen peatland Striegl, 2007; Lamoureux and Lafreniére, 2014; McClelland province in the world. et al., 2014), the key driving environmental factors of particu- late nutrient concentration and stoichiometry in Arctic rivers – permafrost coverage and lakes and forest proportion in the 2 Study site and methods watershed – remain poorly resolved. In this regard, large continental plains such as the Western The rivers were sampled in the WSL, a huge (> Siberian Lowland (WSL), which contains sizeable reservoirs 2 million km2) peatland and forest zone situated in the taiga of frozen and thawed organic carbon, N, P and inorganic nu- forest, forest-tundra and tundra zone. The position of biomes trients (Sheng et al., 2004; Stepanova et al., 2015; Raudina follows the decrease of mean annual air temperature (MAAT) et al., 2017), may be especially useful in assessing environ- from −0:5 ◦C in the south to −9:5 ◦C in the north (Trofimova mental control on particulate nutrient transport to the Arc- and Balybina, 2014). The annual precipitation increases from tic Ocean. A vast amount of frozen peat in this region can 550 mm at the latitude of Tomsk to 650–700 mm at Noyabrsk strongly affect the coastal Arctic system in the event of per- and further decreases to 600 mm at the lower reaches of the mafrost thaw and enhanced export of river suspended matter Taz River. The annual river runoff gradually increases north- (RSM) from the watersheds. Due to the high homogeneity of ward, from 160–220 mm yr−1 in the permafrost-free region the WSL landscape, lithology and topography, one can use to 280–320 mm yr−1 in the Pur and Taz river basins located the natural north–south gradient of the permafrost zone dis- in the discontinuous-to-continuous permafrost zone (Nikitin tribution to assess the direct impact of permafrost conditions and Zemtsov, 1986). The permafrost distribution also follows on river water chemistry. the latitudinal gradient of MAAT and changes from absent, Detailed studies of the dissolved fraction of WSL river wa- isolated and sporadic in the south to discontinuous and con- ters demonstrated several typical features occurring over a tinuous in the north (Baulin et al., 1967). The peat has ac- sizeable gradient of climate and permafrost. In pioneering tively formed since the beginning of the Holocene until the works of Frey and co-authors it was shown that southern freezing of bogs in the Sub-Boreal period (4500–9000 BP). permafrost-free regions export 3 to 4 times greater amounts After that, the rate of peat formation in bog areas decreased of dissolved C, N and P (Frey and Smith, 2005; Frey et (Peregon et al., 2007; Batuev, 2012).
Details
-
File Typepdf
-
Upload Time-
-
Content LanguagesEnglish
-
Upload UserAnonymous/Not logged-in
-
File Pages18 Page
-
File Size-