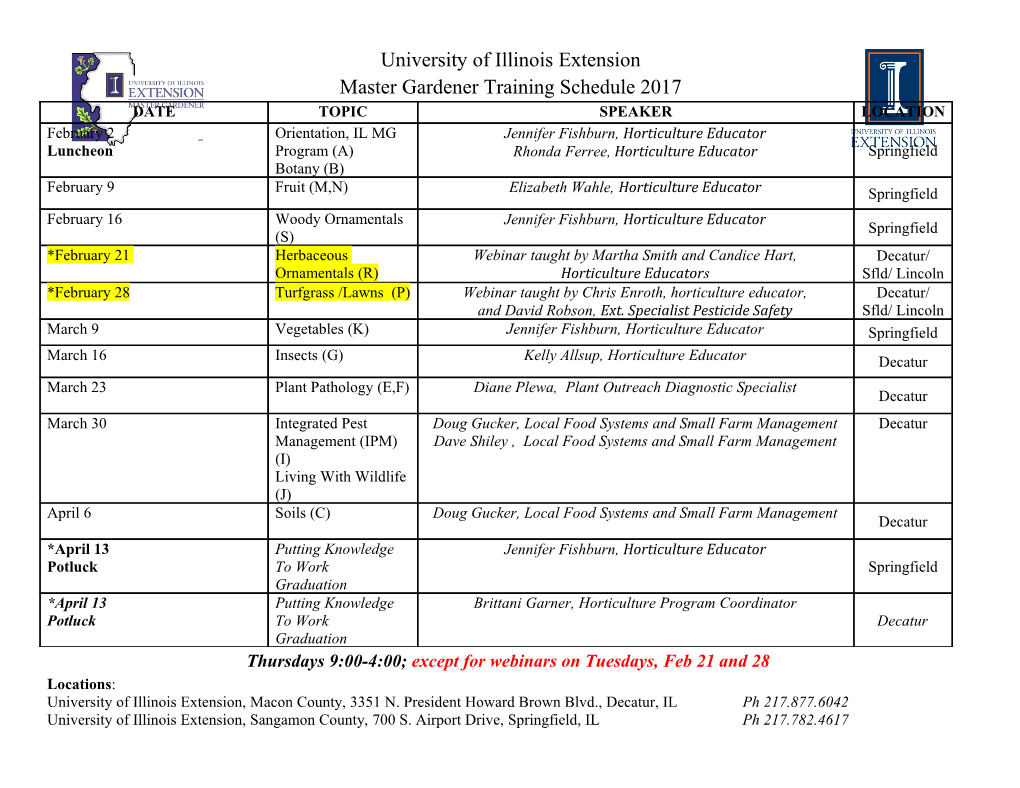
biology Article Comparison of the Photoautotrophic Growth Regimens of Chlorella sorokiniana in a Photobioreactor for Enhanced Biomass Productivity Elvira E. Ziganshina, Svetlana S. Bulynina and Ayrat M. Ziganshin * Department of Microbiology, Institute of Fundamental Medicine and Biology, Kazan (Volga Region) Federal University, 420008 Kazan, Russia; [email protected] (E.E.Z.); [email protected] (S.S.B.) * Correspondence: [email protected]; Tel.: +7-843-233-7881 Received: 29 June 2020; Accepted: 14 July 2020; Published: 16 July 2020 Abstract: Microalgae have a wide industrial potential because of their high metabolic diversity and plasticity. Selection of optimal cultivation methods is important to optimize multi-purpose microalgal biotechnologies. In this research, Chlorella sorokiniana AM-02 that was isolated from a freshwater lake was cultured under various high photosynthetic photon flux density (PPFD) conditions and CO2 gas levels in standard Bold’s basal medium (BBM). Furthermore, a wide range of nitrate 1 levels (180–1440 mg L− ) was tested on the growth of C. sorokiniana. Microalgae growth, pigment concentration, medium pH, exit gas composition, as well as nitrate, phosphate, and sulfate levels were measured during an experimental period. The preferred high PPFD and optimal CO2 levels were 2 1 found to be 1000–1400 µmol photons m− s− and 0.5–2.0% (v/v), respectively. The addition of nitrate 1 ions (up to 1440 mg L− ) to the standard growth medium increased final optical density (OD750), cell count, pigment concentration, and total biomass yield but decreased the initial growth rate at high nitrate levels. Our findings can serve as the basis for a robust photoautotrophic cultivation system to maximize the productivity of large-scale microalgal cultures. Keywords: Chlorella sorokiniana; PPFD; growth; nutrient removal; photobioreactor; productivity; characterization 1. Introduction Microalgae-based biotechnologies have received much attention worldwide, and they are presented as complex processes for purification of air and wastewater as well as for the production of substantial amounts of lipids, polysaccharides, proteins, commercially important pigments (such as lutein and astaxanthin), and other useful products. Pharmaceuticals for various purposes, nutraceuticals, fertilizers, biodiesel, bioethanol, and biogas are obtained from different microalgae species [1–4]. Currently, individual microalgae that are capable of adsorbing or transforming certain pollutants have become excellent candidate species for simultaneously capturing carbon dioxide and removing nutrients, hydrocarbons, pesticides, and cyanide compounds [1,5] as well as for remediation of aquatic ecosystems polluted with heavy metals [6]. The practical application of microalgae metabolic potential can be limited by certain factors, such as photoinhibition and a slow response to different light irradiance, which lead to a low yield of biomass or target products. To increase the cell growth rate, the efficiency of biomass production, and the yield of target products, which means the effectiveness of various microalgae-based technologies, light intensity, photoperiod, temperature, pH, adequacy of macro- and micronutrients, and cultivation regimen (autotrophy, heterotrophy, and mixotrophy) must be thoroughly studied [4,7–9]. Biology 2020, 9, 169; doi:10.3390/biology9070169 www.mdpi.com/journal/biology Biology 2020, 9, 169 2 of 13 Multifarious microalgae species, including algae of the genus Chlorella (Chlorophyta; Trebouxiophyceae; Chlorellales; Chlorellaceae), have been studied in various experimental projects to assess their potential for nutrient bioextraction, bioremediation, and bioenergy processes [7,10]. Species in the genus Chlorella are thought to have high potential for industrial use because of their fast and stable growth characteristics [11–13]. Chlorella sorokiniana is considered to be one of the promising Chlorella species that is used for wastewater treatments as well as for producing valuable products, including lipids, polysaccharides, proteins, and pigments [10,14–16]. Chlorella sorokiniana UTEX 1230 was originally isolated from a stream in Texas in 1951 by Dr. Constantine Sorokin [17], and more detailed information about this species of Chlorella was presented in subsequent research studies [18–20]. C. sorokiniana can grow effectively in a wide temperature range (25–40 ◦C) and under different light intensities [11,18–20]. Representatives of freshwater microalgae have the potential for large-scale cultivation. Researchers have developed various methods of cultivation depending on further practical application goals for the object that is being studied. In photoautotrophic regimens, microalgae cultures assimilate inorganic carbon, and light intensity can significantly affect CO2 assimilation and biomass production [3,10,21]. Among cultivation trends for various industrially attractive microalgae, attention to heterotrophic (substrate dependent) or mixotrophic cultivation (auto- and heterotrophic) is given, which explains the choice with a higher growth rate and lower operating costs of green microalgae [7,11,15]. Although microalgae cultivation under heterotrophic and mixotrophic conditions has some advantages, this process is at risk of contamination with heterotrophic microbes because organic compounds are used as carbon and energy sources [22], and this leads to the release of carbon dioxide as one of the major greenhouse gases. The use of gaseous CO2 as a carbon source by photoautotrophic microalgae contributes to sequestration of carbon dioxide, which is one way to reduce the risks that are associated with global warming [23,24]. Furthermore, when synthesis of the target products is the cell’s physiological response to high light stress, it is important to develop and apply effective strategies for photoautotrophic cultivation [10]. Therefore, additional studies are needed on the characteristics of algae metabolism at different light intensities and CO2 concentrations for each microalgae culture. Moreover, the isolation of new strains with high rates of nitrogen, phosphorus, and sulfur removal is important for the development of new wastewater treatment technologies. Despite recent success in this research area, it is important to develop novel methods and optimize previous methods of growing and harvesting of microalgae so that many attractive species for biotechnology can be grown in a short time and with less corresponding energy use. C. sorokiniana as one of the attractive species for wastewater treatment was selected for the current study. Thus, this study evaluated the optimal high photosynthetic photon flux density (PPFD) conditions and CO2 levels on the growth rate and the ability to utilize important nutrients, such as nitrate, phosphate, and sulfate, by indigenous C. sorokiniana AM-02 that tolerates high irradiance. In addition, based on the optimal growth parameters for C. sorokiniana, the influence of additional nitrogen and sulfur sources on the biomass productivity was investigated. The results presented here offer a new strategy for improving productivity and decreasing resource costs. 2. Materials and Methods 2.1. Isolation and Identification of Microalgal Strain The microalgae culture of Chlorella sorokiniana AM-02 was isolated from a freshwater lake located in Kazan (Russia) in 2018. The isolated strain was made axenic by continuous sub-culturing using Bold’s basal medium (BBM) [25] on agar plates supplemented with ampicillin and kanamycin (10 and 1 50 µg mL− in the medium, respectively). The axenic culture was identified using molecular analysis of the ribulose bisphosphate carboxylase large subunit (rbcL) gene and translational elongation factor Tu (tufA) gene markers. For this, a FastDNA spin kit (MP Biomedicals, Solon, OH, USA) and a FastPrep-24 homogenizer (MP Biomedicals, Solon, OH, USA) were used to extract DNA from microalgae in Biology 2020, 9, 169 3 of 13 accordance with the manufacturer’s protocol. DNA was then quantified with a NanoDrop 2000 spectrophotometer (Thermo Fisher Scientific, Wilmington, DE, USA) and stored at 20 C until use. − ◦ Primers for the rbcL and the tufA genes were selected from published studies and were used to identify microalgae (Table1). Amplicons obtained with these primers were purified using a QIAquick PCR Purification kit (Qiagen, Hilden, Germany) and sequenced on a 3730 DNA Analyzer (Thermo Fisher Scientific, Wilmington, DE, USA) according to the manufacturers’ protocols. Sequencing data was confirmed by BLAST searches in the GenBank’s database and using the BOLD Identification System for the ribulose bisphosphate carboxylase gene. The determined rbcL gene and tufA gene sequences were deposited in the GenBank database under accession numbers MT647139 and MT647140, respectively. Table 1. List of primers used in this study to identify the strain AM-02. Molecular Marker Primers Sequence 5 3 Reference 0 ! 0 rbcL-M379 F GGTTTCAAAGCTYTWCGTGC [26] rbcL (ribulose bisphosphate rbcLFP R GTAAATACCACGGCTACGRTCTT carboxylase large subunit) Fw_rbcl_192 GGTACTTGGACAACWGTWTGGAC [27] Rv_rbcL_657 GAAACGGTCTCKCCARCGCAT tufA (translational tuf A F GGNGCNGCNCAAATGGAYGG [28] elongation factor Tu) tuf A R CCTTCNCGAATMGCRAAWCGC 2.2. Cultivation Conditions Before starting the experiments in a photobioreactor, C. sorokiniana was placed in 250 mL Erlenmeyer flasks containing 30 mL of BBM under sterile conditions to eliminate any contamination. The inoculum was grown with shaking at 120 rpm at +26 ◦C under
Details
-
File Typepdf
-
Upload Time-
-
Content LanguagesEnglish
-
Upload UserAnonymous/Not logged-in
-
File Pages13 Page
-
File Size-