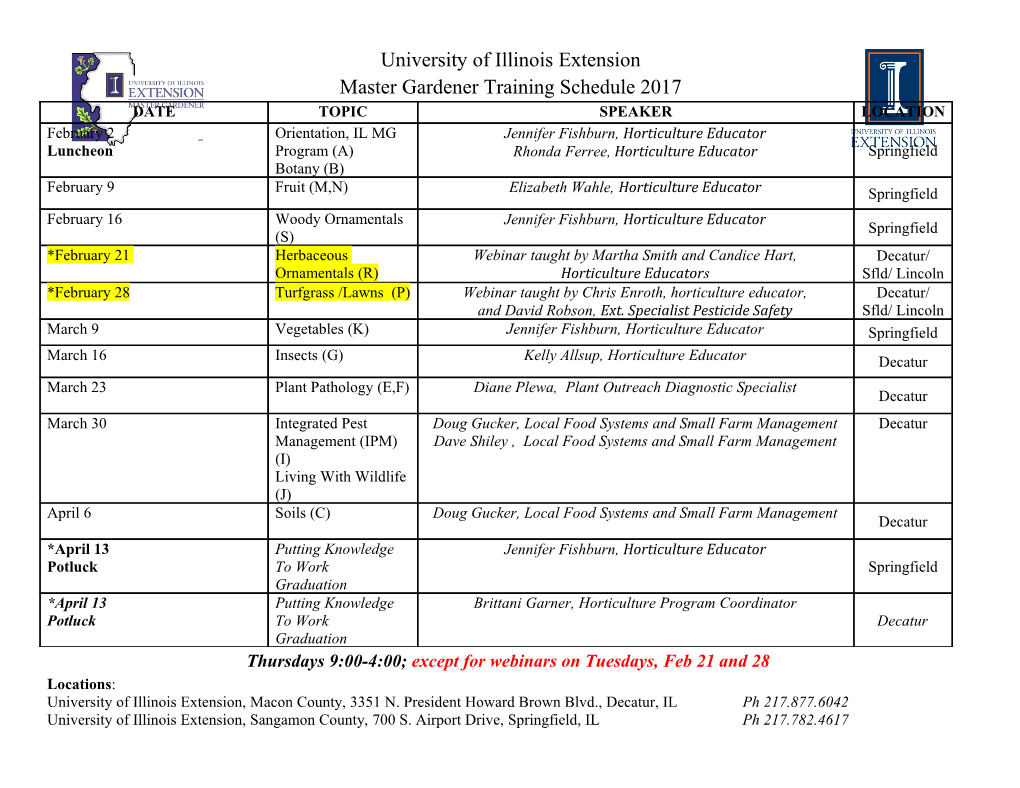
bioRxiv preprint doi: https://doi.org/10.1101/2020.04.22.055384; this version posted January 12, 2021. The copyright holder for this preprint (which was not certified by peer review) is the author/funder, who has granted bioRxiv a license to display the preprint in perpetuity. It is made available under aCC-BY 4.0 International license. Mitofusins Mfn1 and Mfn2 are required in the β-cell to preserve mitochondrial architecture and insulin secretion Eleni Georgiadou1, Charanya Muralidharan2, Michelle Martinez2, Pauline Chabosseau1, Alejandra Tomas1, Fiona Yong Su Wern3, Theodoros Stylianides4, Asger Wretlind5, Cristina Legido-Quigley5,6, Nour Alsabeeh7, Céline Cruciani-Guglielmacci8, Christophe Magnan8, Mark Ibberson9, Isabelle Leclerc1, Yusuf Ali3, Amelia K. Linnemann2, Tristan A. Rodriguez10 and Guy A. Rutter1,3*. 1Section of Cell Biology and Functional Genomics, Division of Diabetes, Endocrinology and Metabolism, Department of Medicine, Imperial College London, London, W12 0NN, UK 2Center for Diabetes and Metabolic Diseases, Indiana University School of Medicine, Indianapolis, IN, 46202, USA 3Lee Kong Chian School of Medicine, Nanyang Technological University, 637553, Singapore 4Loughborough University, Centre of Innovative and Collaborative Construction Engineering, Leicestershire, LE11 3TU, UK 5Systems Medicin, Steno Diabetes Center Copenhagen, 2820, Denmark 6 Institute of Pharmaceutical Science, Kings College London, London, SE1 9NH, UK 7 Kuwait University, Department of Physiology, Health Sciences Center, 13110, Kuwait 8Université de Paris, BFA, UMR 8251, CNRS, Regulation of Glycemia by Central Nervous System, Paris, 75205, France 9Vital-IT Group, SIB Swiss Institute of Bioinformatics, Lausanne, CH-1015,Switzerland 10National Heart and Lung Institute, Imperial Centre for Translational and Experimental Medicine, Imperial College London, London, W12 0NN, UK *Address correspondence to Professor Guy A. Rutter, [email protected], +44 20 759 43340 1 bioRxiv preprint doi: https://doi.org/10.1101/2020.04.22.055384; this version posted January 12, 2021. The copyright holder for this preprint (which was not certified by peer review) is the author/funder, who has granted bioRxiv a license to display the preprint in perpetuity. It is made available under aCC-BY 4.0 International license. Summary Mitochondrial glucose metabolism is essential for the initiation of insulin release from pancreatic β-cells. Fusion of mitochondria is supported by mitofusin 1 (MFN1) and mitofusin 2 (MFN2). Whether this process is important for glucose sensing by β-cells is unclear. Here, we generated mice with β-cell-selective, adult-restricted deletion of Mfn1 and Mfn2 (Mfn1/2-KO), and explored the impact on insulin secretion and glucose homeostasis. Mfn1/2-KO mice displayed higher glycaemia and a >five-fold decrease in plasma insulin post-intraperitoneal glucose injection. Mitochondrial length, glucose-induced hyperpolarization, ATP synthesis and Ca2+ accumulation were significantly reduced in Mfn1/2-KO mouse islets. Ca2+ dynamics and mitochondrial membrane potential changes were also suppressed in vivo. Defective glucose- stimulated insulin secretion in islets isolated from Mfn1/2-KO mice was nevertheless normalised by the addition of GLP-1 receptor agonists. Mitochondrial fusion and fission cycles are thus essential in the β-cell to maintain normal mitochondrial bioenergetics and glucose sensing both in vitro and in vivo. Keywords: Type 2 diabetes, pancreatic β-cell, mitochondrial dysfunction, mitofusins, glucose stimulated insulin secretion, Ca2+ dynamics, incretins, GLP-1, GIP, Exendin- 4. 2 bioRxiv preprint doi: https://doi.org/10.1101/2020.04.22.055384; this version posted January 12, 2021. The copyright holder for this preprint (which was not certified by peer review) is the author/funder, who has granted bioRxiv a license to display the preprint in perpetuity. It is made available under aCC-BY 4.0 International license. Introduction Mitochondria are often referred to as the powerhouses or, more recently, as the “chief executive organelles” (CEO) of the cell, generating most of the energy required to sustain normal function (Anderson, Jackson et al. 2019). Mitochondria are responsible for NAD and NADH production via the Krebs cycle, oxidative phosphorylation, ATP synthesis, fatty acid oxidation and gluconeogenesis, and mitochondrial DNA (mtDNA) distribution. They also contribute to the regulation of apoptosis and their own turnover via mitophagy (Chandhok, Lazarou et al. 2018). Mitochondrial oxidative metabolism plays a pivotal role in the response of pancreatic β-cells to stimulation by glucose and other nutrients (Panten and Ishida 1974, Maechler and Wollheim 2001, Rutter, Pullen et al. 2015). Thus, as the blood glucose levels increase in the blood, enhanced glycolytic flux and oxidative metabolism in β- cells lead to an increase in ATP synthesis, initiating a cascade of events which involve + the closure of ATP-sensitive K (KATP) channels (Rorsman and Ashcroft 2018), plasma membrane depolarisation and the influx of Ca2+ via voltage-gated Ca2+ channels (VDCC). The latter mechanism, along with other, less well defined amplifying signals (Henquin 2000), drive the biphasic release of insulin (Rutter, Pullen et al. 2015). The possibility that changes in mitochondrial function in these cells may contribute to declining insulin secretion and to type 2 diabetes (T2D) has been the subject of extensive investigation (Supale, Li et al. 2012). Reduced glucose-stimulated insulin secretion (GSIS) in β-cells, alongside altered mitochondrial function, dynamics and morphology, were observed in diabetic models (Del Guerra, Lupi et al. 2005, Mulder and Ling 2009, Rutter GA, Georgiadou E et al. 2020). 3 bioRxiv preprint doi: https://doi.org/10.1101/2020.04.22.055384; this version posted January 12, 2021. The copyright holder for this preprint (which was not certified by peer review) is the author/funder, who has granted bioRxiv a license to display the preprint in perpetuity. It is made available under aCC-BY 4.0 International license. Besides synthesizing ATP, mitochondria are also one of the main producers of rative oxygen species (ROS) in the cell, inducing oxidative stress and tissue damage (Szendroedi, Phielix et al. 2012). Mitochondrial dysfunction in type 2 diabetic or obese patients suffering from hyperglycaemia was shown to be directly linked with lowered ATP levels and mitochondrial content as well as the development of insulin resistance (Rovira-Llopis, Banuls et al. 2017). Additionally, mitochondrial DNA (mtDNA) variations in human populations are associated with increased or decreased risk of T2D (van den Ouweland, Maechler et al. 1999), while in animal models, alterations in β-cell mtDNA lead to reduced insulin secretion, hyperglycaemia and β-cell death (Haythorne, Rohm et al. 2019). The multifaceted roles of mitochondria in the cell are associated with an equally variable morphology. Under normal physiological conditions, these organelles repetitively undergo fusion and fission cycles which are essential for their quality control and adaptation to energetic demands (Wada and Nakatsuka 2016). Thus, highly inter-connected mitochondrial networks allow communication and interchange of contents between mitochondrial compartments, as well as with other organelles such as the ER (Rutter and Rizzuto 2000). These networks exist interchangeably with more fragmented structures, displaying more “classical” mitochondrial morphology, with the balance between the two influenced by external stimuli and metabolic demands (Westermann 2012). In many cell types, including insulinoma-derived INS1 cells, a pro-fused state is often observed during starvation where energy production is required for cytoprotection and resistance to apoptosis. The opposite pertains when cells are exposed to nutrient overload in obesity or T2D (Liesa and Shirihai 2013) which can stimulate mitochondrial fission and uncoupled respiration (Liesa and 4 bioRxiv preprint doi: https://doi.org/10.1101/2020.04.22.055384; this version posted January 12, 2021. The copyright holder for this preprint (which was not certified by peer review) is the author/funder, who has granted bioRxiv a license to display the preprint in perpetuity. It is made available under aCC-BY 4.0 International license. Shirihai 2013), but is critical for the elimination of damaged mitochondria by mitophagy (Wai and Langer 2016). Over the past two decades, considerable light has been shed upon the means through which mitochondrial dynamics are controlled at the molecular level. The mitofusins MFN1 and MFN2, homologues of the D. melanogaster fuzzy onions (fzo) and mitofusin (dmfn) gene products (Hwa, Hiller et al. 2002), are key mediators of outer mitochondrial membrane (OMM) fusion. Whilst optic atrophy protein 1 (OPA1) controls inner mitochondrial membrane (IMM) fusion, dynamin related protein 1 (DRP1) is responsible for mitochondrial fission (Yisang Yoon 2011). Other regulators include FIS1, mitochondrial fission factor (MFF) and MiD49/51 (Serasinghe and Chipuk 2017). In pancreatic β-cells, defects in mitochondrial structure and the accumulation of damaged or depolarised organelles are associated with the development of diabetes in patients and animal models (Wada and Nakatsuka 2016). Changes in mitochondrial fusion and fission dynamics are observed in the pancreatic β-cell in animal models of diabetes (Higa, Zhou et al. 1999, Supale, Li et al. 2012, Gao, Li et al. 2014), and patients with T2D and obesity exhibit smaller
Details
-
File Typepdf
-
Upload Time-
-
Content LanguagesEnglish
-
Upload UserAnonymous/Not logged-in
-
File Pages58 Page
-
File Size-