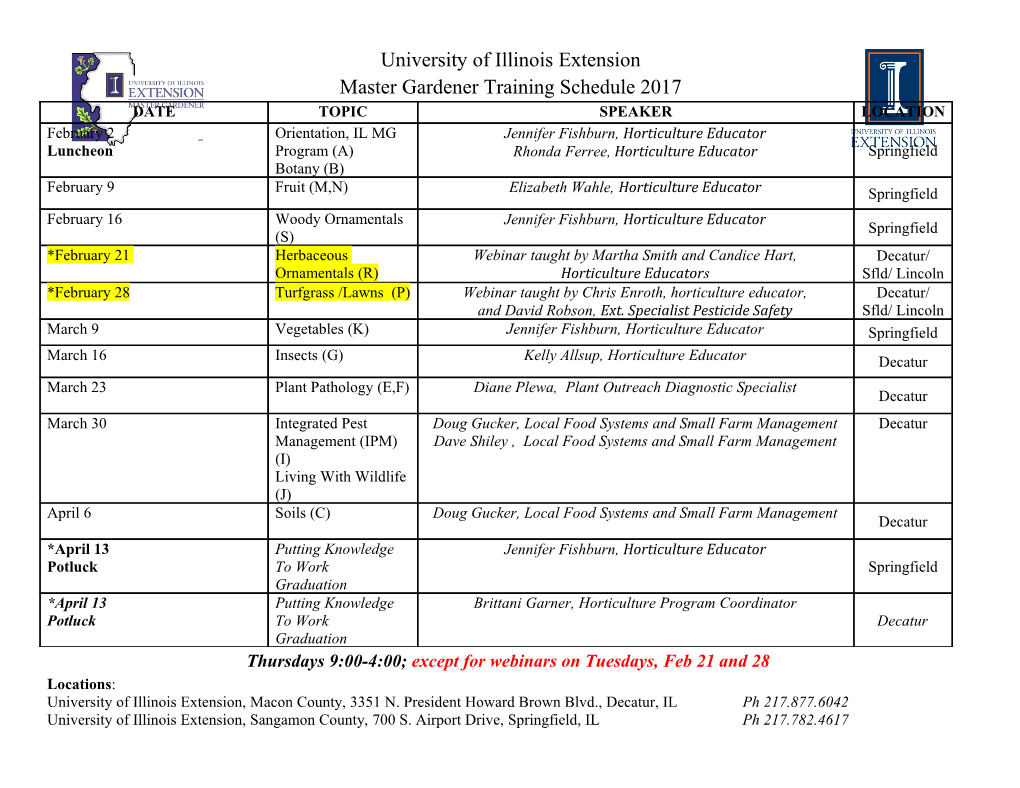
Molecular Mechanisms Regulating Mammalian Forebrain Development By David Chun Cheong Tsui A thesis submitted in conformity with the requirements for the degree of Doctor of Philosophy Institute of Medical Science University of Toronto © Copyright by David Chun Cheong Tsui (2013) Title: Molecular Mechanisms Regulating Mammalian Forebrain Development Name: David Chun Cheong Tsui Degree: Doctor of Philosophy, 2013 Department: Institute of Medical Sciences, University of Toronto ABSTRACT While the extrinsic factors regulating neurogenesis in the developing forebrain have been widely studied, the mechanisms downstream of the various signaling pathways are relatively ill-defined. In particular, we focused on proteins that have been implicated in cognitive dysfunction. Here, we ask what role two cell intrinsic factors play in the development of two different neurogenic compartments in the forebrain. In the first part of the thesis, the transcription factor FoxP2, which is mutated in individuals who have specific language deficits, was identified to regulate neurogenesis in the developing cortex, in part by regulating the transition from the radial precursors to the transit amplifying intermediate progenitors. Moreover, we found that ectopic expression of the human homologue of the protein promotes neurogenesis in the murine cortex, thereby acting as a gain-of-function isoform. In the second part of the thesis, the histone acetyltransferase CREB-binding protein (CBP) was identified as regulating the generation of neurons from medial ganglionic eminence precursors, similar to its role in the developing cortex. But CBP also plays a more substantial role in the expression of late interneuron markers, suggesting that it is continuously required for the various stages of neurogenesis at least in the ventral neurogenic niche. Finally, similar to cortical precursors, the function of CBP in the ventral forebrain precursors is also dependent on histone acetylation. Together, these studies shed light on some of the key intrinsic players that regulate the differentiation of neural precursors in the embryonic murine forebrain, and they also suggest potential mechanisms for the pathogenesis of various cognitive dysfunctions. ii ACKNOWLEDGEMENTS I am grateful to my family and everyone who supported me during my PhD training. In particular, I thank Freda and David for teaching me how to focus on important scientific questions, critically think about experimental results and to be scientifically creative. In addition, they helped me to significantly improve my presentation and writing skills. Their guidance and support were valuable for my training experience and I will always be grateful for them pushing me to reach success. I also thank Derek van der Kooy, my committee member and also my previous research supervisor, for introducing me to the world of science and also pushing me to think outside the box. My experience in Derek’s laboratory had a great influence on my decision to pursue a scientific career. Many of my colleagues in the lab have been crucial role models and people to exchange ideas with during my journey. In particular, I am grateful to Sarah Burns for teaching me the skills of in utero electroporation and also helping me to carry out some of the in utero electroporations in my projects; Jing Wang for teaching me the skills of cell culturing and critical thinking; John Vessey and Hideaki Tomita for assisting me in some of my experiments as well as their intellectual input. I’d also like to thank Joseph Anthony, Jeff Biernaskie, Sagar Dugani, Masashi Fujitani, Denis Gallagher, Andree Gauthier-Fisher, Hiroyuki Jinno, Adam Johnston, Andreea Norman, Milijana Vojvodic, Ian Weaver, and Mark Zander for their discussions, and the rest of the Miller and Kaplan labs. Finally, I thank the MD/PhD office for their advice and support over the years: Director Dr. Norman Rosenblum, Sandy McGugan, and past-director Dr. Mel Silverman. I’d also like to thank my MD/PhD classmates for their input and discussion during the seminars. iii PUBLICATION AND AUTHOR CONTRIBUTIONS Chapter 4 was accepted for publication in the Journal of Neuroscience. David Tsui performed all experiments of the chapter except for the experiments listed below, and co-wrote the manuscript with Freda Miller and David Kaplan. John Vessey performed and analyzed experiments in figure 4.4F, 4.4J, 4.4K and 4.4L. Sarah Burns performed in utero electroporations for some of the in vivo experiements. Both John Vessey and Hideaki Tomita provided advice. Freda Miller and David Kaplan helped design experiments, provided support, advice and guidance. This work was supported by Canadian Institutes of Health Research (CIHR) Grant MOP 13958 from the CIHR to Freda Miller and David Kaplan. David Tsui was supported by a CIHR CGS-D scholarship. David Tsui performed all the experiments in Chapter 5. iv TABLE OF CONTENTS ABSTRACT ii ACKNOWLEDGEMENTS iii PUBLICATION AND AUTHOR CONTRIBUTIONS iv TABLE OF CONTENTS v LIST OF FIGURES viii LIST OF ABBREVATIONS ix CHAPTER 1: INTRODUCTION 1 1.1 Neurogenesis in the developing cortex 2 1.1.1 Overview of murine cortical neurogenesis 4 A) Transition from neuroepithelial stem cells to radial precursor cells 4 B) Direct neurogenesis from radial precursor cells 6 C) Indirect neurogenesis via generation of basal progenitors 10 1.1.2 Extrinsic and intrinsic factors regulating neurogenesis 14 A) Extracellular signaling – ligands and receptors 14 B) Signaling cascades 25 C) Transcription factors 26 D) Epigenetic factors 32 1.2 Neurogenesis in the developing ventral forebrain 34 1.2.1 Overview of ventral forebrain neurogenesis 34 A) Generation of interneurons and basal forebrain cholinergic neurons 35 B) Tangential migration of cortical interneurons 38 C) Origin of interneuron subtypes 39 1.2.2 Extrinsic and intrinsic factors regulating neurogenesis 40 A) Regulation of interneuron generation 40 1.3 CREB binding protein (CBP) 44 v 1.3.1 CBP and Rubinstein-Taybi syndrome 44 1.3.2 Role of CBP in muscle and hematopoetic differentiation 45 1.3.2 Role of CBP in neural differentiation 46 1.4 Forkhead box protein P2 (FoxP2) 46 1.4.1 FoxP2 and specific language impairment 46 1.4.2 Mouse models of FoxP2 mutants 47 CHAPTER 2: OVERALL HYPOTHESIS AND AIMS 57 CHAPTER 3: MATERIALS AND EXPERIMENTAL PROCEDURES 59 Animals 59 Primers and Plasmids 59 Cortical precursor cell cultures 60 Medial ganglionic eminence precursor cell cultures 61 In utero electroporation 62 Immunocytochemistry and histological analysis 62 Western blot analysis 63 Flow cytometry 64 HEK-293 experiments 65 RT-PCR 65 Microscopy and quantification 65 Statistics 67 vi CHAPTER 4: FOXP2 PROMOTES NEUROGENESIS IN THE MAMMALIAN CORTEX Abstract 68 Introduction 69 Results 70 Figures 83 Discussion 102 CHAPTER 5: CBP REGULATES INTERNEURON DIFFERENTIATION AND MATURATION 107 Abstract 107 Introduction 108 Results 110 Figures 117 Discussion 127 CHAPTER 6: DISCUSSION and FUTURE DIRECTIONS 131 CHAPTER 7: REFERENCES 145 vii LIST OF FIGURES Figure 1.1: Evolution of cortical development 49 Figure 1.2: Receptor tyrosine kinase (RTK) activation of four different pathways 51 Figure 1.3: Wnt binding activates transcription of genes that mediate precursor maintenance 53 Figure 1.4: Parallel pathways regulate neurogenesis in the medial ganglionic eminence 55 Figure 4.1: Expression of FoxP2 in embryonic cortical precursors 83 Figure 4.2: FoxP2 knockdown decreases neurogenesis in the embryonic cortex in vivo 86 Figure 4.3: FoxP2 knockdown increases radial precursors at the expense of intermediate progenitors in the embryonic cortex 89 Figure 4.4: FoxP2 knockdown increases radial precursors at the expense of intermediate progenitors and neurons, and this is reversed by coincident expression of human FoxP2 92 Figure 4.5: Ectopic expression of human but not murine FoxP2 in the murine embryonic cortex enhances the genesis of intermediate progenitors and neurons 95 Figure 4.6: Ectopic expression of human but not mouse FoxP2 promotes genesis of intermediate progenitors and neurons 98 Figure 4.7: The KE family FoxP2 mutation acts as a dominant-negative with regard to embryonic cortical neurogenesis 100 Figure 5.1: Expression of CBP in medial ganglionic eminence (MGE) precursors 117 Figure 5.2: CBP knockdown in MGE precursors reduces neurogenesis without affecting precursor survival or proliferation 119 Figure 5.3: CBP knockdown in MGE precursors reduces the percentage of neurons expressing mature interneuron markers 121 Figure 5.4: CBP knockdown in MGE precursors reduces the percentage of neurons expressing mature interneuron markers 123 Figure 5.5. The interneuron development deficit caused by CBP knockdown is rescued by WT-CBP and the HDAC inhibitor Trichostatin A (TSA) 125 Figure 6.1: Schematic of the proposed pathway of FoxP2 in cortical neurogenesis 141 Figure 6.2: Schematic of the protein structure of CBP and p300 143 viii LIST OF ABBREVIATIONS AF-6 ALL-1 fusion partner from chromosome 6 APC Adenomatous polyposis coli aPKC Atypical protein kinase C Arx Aristaless-related homeobox protein ASPM Abnormal spindle-like microcephaly-associated protein BDNF Brain-derived neurotrophic factor BLBP Brain-lipid-binding protein BMP Bone morphogenetic protein BrdU Bromodeoxyuridine BSA Bovine serum albumin C/EBP CCAAT/enhancer-binding proteins CADM1 Cell adhesion molecule 1 CBF1 C repeat binding factor 1 CBP CREB binding protein CC3 Cleaved caspase 3 CGE Caudal ganglionic eminence ChAT Choline acetyltransferase ChIP Chromatin immunoprecipitation CMV Cytomegalovirus
Details
-
File Typepdf
-
Upload Time-
-
Content LanguagesEnglish
-
Upload UserAnonymous/Not logged-in
-
File Pages192 Page
-
File Size-