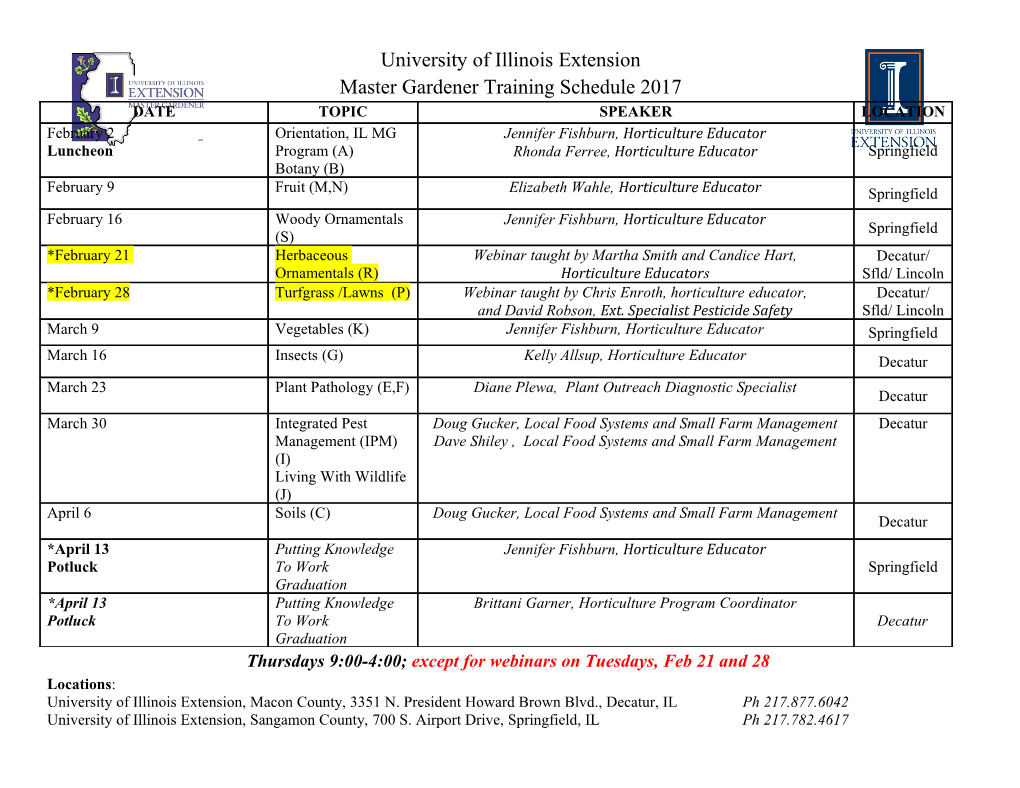
COMPACT HEAT EXCHANGERS FOR MOBILE CO2 SYSTEMS A. Hafner SINTEF Energy Research Refrigeration and Air Conditioning Trondheim (Norway) ABSTRACT The natural refrigerant carbon dioxide (CO2) with all its advantages offers new possibilities of efficient heating and cooling at different climates. In reversible air conditioning systems the capacity and efficiency in cooling mode is seen to be most important. The efficiency of the reversible interior heat exchanger depends on the design of the heat exchanger. Efficiency reduction is among other factors caused by refrigerant- side pressure drop, heat conduction, refrigerant- and air maldistribution. Uniform air temperatures at the outlet of the heat exchanger are an important aspect regarding comfort and control of the mobile HVAC system. A prototype CO2 system with a separator, refrigerant pump and a single pass heat exchanger was tested under varies conditions. The tests were performed at low compressor revolution speed i.e. idle conditions. Compared with a baseline heat exchanger of equal size, the use of an external operated pump to circulate the refrigerant through the heat exchanger, increased the cooling capacity at equal pressure levels by up to 14 %. At equal cooling capacities the COP increased by up to 23 %. Airside temperature distribution was more uniform with this way of operating the system. 1 INTRODUCTION Several authors for example Hrnjak et al 2002, Nekså et al. 2001 describe CO2 systems where flash gas was bypassed the evaporator and thereafter reunified with the leaving gas of the evaporator. With such a system concept only liquid refrigerant enters the evaporator which has an positive effect on distribution of the refrigerant into 20000 the microchannels. Studies performed at G = 190 G = 280 SINTEF Energy Research (Figure 1.1) show G = 380 G = 570 a dramatic reduction on heat transfer 15000 coefficients inside microchannel tubes at 1 - higher vapour fraction and mass flux. On the K 2 other hand the heat transfer coefficients at - 10000 m lower vapour fraction had been in the range W of 12.000 W/m2K nearly independent of the h, mass flux. This was the basis for this study of 5000 an evaporator inside an entire CO2 system, where an external pump was used to circulate the refrigerant through the evaporator. In this 0 case the refrigerant side heat transfer should 0.00.20.40.60.81.0 not drop and the capacity of the heat vapour fraktion exchanger should increase. Figure 1.1 Influence of the mass flux on the heat transfer coefficient, from Pettersen. 2002. IIF-IIR – Commission B1, B2, E1 and E2 – Guangzhou, China - 2002 2 MEASUREMENT EQUIPMENT The test facility consists of two climate chambers with wind tunnels containing the prototype heat exchangers, and a compressor drive arrangement. (Hafner, 2000). The refrigerant circuiting around the interior heat exchanger was modified and a gear pump was used to circulate the liquid CO2 from a separator. The baseline evaporator was a prototype CO2 microchannel heat exchanger with 2 rows and 7 passes. This was a unit built from extruded microchannel tubes of 41mm width (Pettersen et al., 1998). To get a single pass heat exchanger with reduced pressure drop in mind, the connectors of a similar heat exchanger (2 rows – 6 passes, 10% reduced front surface) was connected in a way to get a equally bottom fed 2row-1pass heat exchanger. Exterior HX A made-to order sight-glass was used as the liquid separator / low- pressure receiver in the CO2 circuit. 3 r The internal volume was 1 dm . The o s separator/receiver, the gear pump and s e r the mass flow meter were located p m inside the climate chamber. The Co revolution speed of the gear pump was controlled with a 12V DC power High side pressure Suction line HX control valve supply. The maximum refrigerant mass flow rate of the pump at 50 bar evaporation pressure was around 3.2 kg/min. In front of the separator a video camera was installed to observe r the liquid level inside. to a r a p The compressor for the test 3 Se program was a 20.7 cm reciprocating prototype machine The prototype CO2 microchannel parallel-flow heat Evaporator exchanger served as the exterior heat exchanger. Gear The suction line heat pump exchanger used was an extruded aluminium profile made by Hydro Mass flow meter Aluminium. The total length of the heat exchanger was 1.5 m and it was m bent into a U-shape, so the inlet and outlet were side by side. A manual metering valve was Figure 2.1 Flow circuit of the CO2 prototype system used as the expansion device during the test series. A grid of 20 thermocouples was installed 10 cm behind the interior heat exchanger to measure temperature distribution on the air side. IIF-IIR – Commission B1, B2, E1 and E2 – Guangzhou, China - 2002 3 EXPERIMENTS AND RESULTS Results from earlier measurements of the 2row-7pass heat exchanger were used as baseline for the comparison. The baseline CO2 system included a ‘conventional’ low-pressure receiver. In the same CO2 system the 2row-7pass heat exchanger was replaced by a 2row-1pass heat exchanger (identical tubes, fins and headers), a liquid separator and an external controlled refrigerant pump (Fig.2.1) were added to perform the test program. The performance of the 2row-1pass was studied at varying refrigerant mass flow rates and a test with natural refrigerant circulation was performed. Most of the tests were performed at an air inlet temperature to the interior heat exchanger of 41 °C at a relative humidity of 40 %. The air mass flow rate through the interior heat exchanger was kept to 8.1 kg/min (420m3/h). During most of the tests the high side pressure was controlled to be at 100 bar, except the test where the optimum high side pressure was investigated. Under these COP-tests, the airside velocity of the exterior heat exchanger was 2.4 m/s at a temperature of 39 °C. The baseline test data where taken with a high performance gascooler, therefore the inlet vapour fraction to the evaporator was x=0.2, see Figure 3.1. Equal airside temperature reduction at: • equal air mass flow rates, • equal air face velocities • equal evaporation pressure were applied in the direct comparisons between the heat exchangers. baseline 1 pass 3.2 2.6 2 kg/min 120 120 110 110 100 Evaporator 100 Evaporator ] ] r inlet outlet inlet outlet ar a b b 90 90 [ [ e e r r 80 u 80 s ssu es e 70 70 r r P P 60 60 50 50 40 40 500 600 700 800 500 600 700 800 Specific enthalpy [kJ/kg] Specific enthalpy [kJ/kg] Figure 3.1 Process values of the Figure 3.2 Process values of the pump systems in a p, h-diagram. Equal air side circulating system in a p, h-diagram. Equal air capacity at 8.1 kg/min air mass flow rate. side capacity at 8.1 kg/min air mass flow rate and varying refrigerant mass flow rates. A refrigerant liquid overfeeding factor through the evaporator of 2 was reached with the gear pump at maximum revolution speed; see the leftmost columns of Figure 3.3. The refrigerant mass flow rate through the compressor was in the same range as for the baseline test at 1.55 kg/min during the tests at equal air face velocity and equal air mass flow rate through the evaporator, while it was 15% higher for the test at equal evaporation pressure. IIF-IIR – Commission B1, B2, E1 and E2 – Guangzhou, China - 2002 The refrigerant side pressure drop was 3.6 times lower for the tests with pump circulation even with twice the refrigerant mass flow rate through the evaporator (3.1 kg/min) ref. mass flow rate, compressor ref. mass flow rate, evap. pressure drop vapour fraction, evap. outlet 4.0 0.8 vapour fraction separator inlet: 0.16 0.16 0.2 0.48 0.47 0.46 0.34 3.5 0.7 ] [- . n ] o n i ti 3.0 0.6 c m / a g fr r [k u o te 2.5 0.5 p a r w o 2.0 0.4 ] / Va fl r s a s a [b 1.5 0.3 p o t m r n a d r e e r g u i 1.0 0.2 s fr s e e R Pr 0.5 0.1 0.0 0.0 y ) ) ) it te f f f c. ine e e re ir el oc a l_p0 (r (r ( c l r n n n . e i f bas low m e f equa /mi /mi / r s ide v s g g al s kg pas r s a .2 k .6 k 2 ur i 3 2 7 r m nat q_a i e q._a m air, interior = 8.1 kg/min; t air, interior = 41 °C (40% rel. hum.) e Figure 3.3 Refrigerant mass flow rate (through evaporator dark filled bar +amount through compressor lighter filled bar), refrigerant side pressure, vapour fraction at inlet to separator and outlet from the evaporator for the different test series. Figure 3.4 shows the effect of liquid overfeeding on the evaporation pressure. For the same capacity it raised from 50 bar for the baseline system to 54 bar for the tests at equal air face velocity and air mass flow rate. When keeping the evaporation pressure at the same level the cooling capacity increased by 14% at an equal amount of air through the evaporators.
Details
-
File Typepdf
-
Upload Time-
-
Content LanguagesEnglish
-
Upload UserAnonymous/Not logged-in
-
File Pages8 Page
-
File Size-